Abstract
Studies on hydrogen and CO2 storage in the subsurface are vital for advancing clean energy and climate change mitigation. They help optimize underground hydrogen storage for balancing energy supply and demand and improve carbon capture and storage (CCS) techniques to trap CO2, reducing atmospheric levels securely. These studies can ensure the geotechnical stability of storage sites, minimizing leakage risks, while enhancing our understanding of the storage capacity in porous media like sandstone, leading to safer and more efficient long-term storage solutions. This study explores hydrogen and CO2 storage on a laboratory scale to determine how these gases behave in porous sandstone media under varying pressure conditions. Further, this study typically investigates to understand how well the porous media can store and retain gases. The study also examines the mechanisms of trapping (capillary and residual trapping) of hydrogen and CO2, which is essential for long-term underground storage.
1. Introduction
Hydrogen (H2) energy is gaining popularity as a future energy source. The current changing climate is mainly caused by carbon emissions globally [1-3]. To reduce this, the use of H2 is highly effective that can spark the energy sector with zero carbon emissions and increase energy flexibility [4]. The IPCC’s 1.5 °C Report states that hydrogen must play a significant role as a fuel substitute to limit global warming [5]. The European Union (EU) wants to be a low-carbon economy by 2050, with the implementation of hydrogen-supported technologies in the energy sector. Compared to its 1990s level, the EU aims to reduce carbon emissions by at least 40 % by 2030 [6], raise the percentage of renewable energy generation to at least 32 %, and enhance energy efficiency by at least 32.5 %. By 2050, a completely carbon-free energy system is the ultimate objective of the EU [5]. As a member of the EU, Lithuania (the Baltic Sea region country) has the potential and is striving to play a greater role in the development and mobilization of hydrogen storage in Europe.
It has also led to creation of H2 platform under the Ministry of energy with aim of fostering an environment to promote development of H2 technologies in Lithuania. Although H2 is a very efficient fuel it has many challenges associated with its use. H2 being a very light gas requires large storage volume, therefore, for the effective adoption of H2 based technologies in Lithuania, questions of H2 storage have to be addressed. Underground gas storage has been routinely carried out in Europe and even in Lithuania methane is stored in summer in underground (Inčukalns UGS) formation, in Latvia, for use in winter period. Depleted gas field, oil fields and saline aquifers offers possibility to store large volumes of gas and it's possible to use these underground porous formations for storing large quantities of hydrogen. The depleted hydrocarbon reservoirs and deep saline aquifers in Lithuanian basin have been investigated for CO2 storage in past [7], these reservoirs are equally good for storing hydrogen as well.
Therefore, major aim of this study is to conduct a lab-scale experiment to assess the hydrogen flow behavior in porous media under a controlled system. The specific objectives are a) To examine the effectiveness of physical and chemical trapping mechanisms that prevent gas leakage. b) To study the movement and retention of gases in porous and permeable sandstone media.
2. Experiment methodology
The experimental setup for gas injection in a porous media (sandstone) was constructed using a properly engineered tank to assure safety and accuracy in studying hydrogen and CO₂ storage. The experimental setup typically consists of a tank, with pressure and temperature monitoring sensors for data collection. The tank was made of a 20 mm thick acrylic sheet, which was chosen for its longevity and robustness. The tank’s dimensions, 15×15×5 inches, was designed particularly to create a controlled environment that is manageable in size while being large enough to imitate real subsurface conditions. The top and bottom of the tank were made from steel material because of its high tensile strength and durability under pressure. The process began with the selection and preparation of layering materials including sandstone, gravel, fine sand, and clay.
This systematic approach contributes to a better understanding of hydrogen flow behavior. Also, we employed visualization techniques such as a dye (Bromothymol blue) tracer to observe fluid distributions within the sandstone pores. Bromothymol blue (BTB) is a pH-sensitive dye that changes color in response to changes in the pH of the surrounding environment. In alkaline conditions (pH > 7.6), BTB is blue while in neutral conditions (around pH 7), BTB is green and in acidic conditions (pH < 6.0), BTB turns yellow. When CO₂ introduced into a media saturated with water/brine, it reacts with the water/brine and formed carbonic acid (H2CO3), and further dissociated into hydrogen ions (H⁺) and bicarbonate ions (HCO3-), resulting in a lower pH. In this case, when CO2 is introduced, the shift in pH towards acidic conditions forces the equilibrium towards the yellow form of BTB, indicating movement of gases inside the tank:
CO2 (g)+H2O (l)→ H2CO3 (aq).
H2CO3 (aq)→H⁺ (aq)+ HCO3- (aq).
3. Results
Initial trial examinations revealed that injecting methylene blue dye mixed with brine at a constant rate primarily tested the mechanical performance of the apparatus. After introducing compressed air at around 0.5 bar of pressure, an abrupt crack emerged, causing the clay layer to be displaced from its original position. The sandstone layer, which was 5-6 inches deep, was unaffected by this injection, however, the 3-inch clay layer was displaced, indicating that the pressure can cause disturbance within the layers. The lessons learnt from case 1 (Fig. 1) was applied to the subsequent experimental cases 2 and 3.
Fig. 1Case 1-compressed air injection during trial phase of experiment
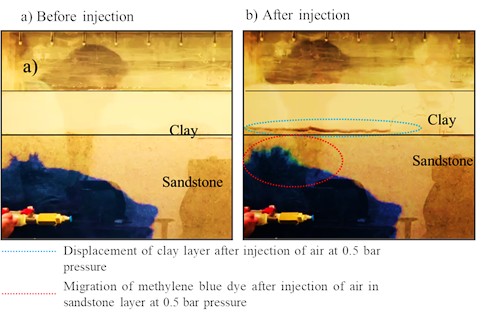
4. Conclusions
The movement of hydrogen and CO2 through the sandstone media was observed from left to right, showing that the porous structure of the sandstone facilitated gas migration. This confirms that sandstone acts as a permeable medium, allowing gases to flow through its interconnected pores. The gases are trapped in the center of the tank, just below the clay layer. This behavior indicates that while sandstone allows gas flow, it relies on an overlying impermeable layer (such as clay) to trap the gases and prevent further vertical movement. This demonstrates the effectiveness of porous sandstone media for storing gases when combined with a sealing layer. As gas injection continued, pressure gradually built up within the tank, reaching a maximum of 0.49 bar. At this point, the porous sandstone media still retained the gas without showing signs of leakage through the geological formation itself. When the system pressure exceeded 0.49 bar, the tank expanded, and leakage occurred. However, the leakage was not through the sandstone or clay, but rather from the top of the system, indicating that while the sandstone media could handle pressure up to a certain point, the structural containment of the system was compromised. Importantly, the clay layer, which was acting as a cap rock, did not fracture even under increased pressure. This behavior suggests that the sandstone below can store gases effectively if paired with an impermeable layer like clay, which prevents vertical gas escape and ensures containment within the storage formation.
Overall, the study shows that while sandstone media is effective in allowing gas movement and storage, the success of such storage depends heavily on the overlying sealing layer and overall system pressure management.
References
-
N. S. Muhammed, B. Haq, D. Al Shehri, A. Al-Ahmed, M. M. Rahman, and E. Zaman, “A review on underground hydrogen storage: Insight into geological sites, influencing factors and future outlook,” Energy Reports, Vol. 8, pp. 461–499, Nov. 2022, https://doi.org/10.1016/j.egyr.2021.12.002
-
A. Verma, S. Malik, and M. Pal, “Feasibility of UHS in Baltic basin: a case study of Lithuanian reservoir in diverse geological settings,” in 5th EAGE Global Energy Transition Conference and Exhibition (GET 2024), Vol. 2024, No. 1, pp. 1–5, Jan. 2024, https://doi.org/10.3997/2214-4609.202421080
-
A. Verma, M. Pal, and S. Malik, “Unlocking hydrogen potential from depleted hydrocarbon reservoirs in Lithuania: a sustainable approach,” ECMOR 2024, Vol. 2024, No. 1, pp. 1–11, Jan. 2024, https://doi.org/10.3997/2214-4609.202437038
-
V. Karaliūtė and M. Pal, “An overview of Baltic carbon forum conference 2022,” Advances in Carbon Capture Utilization and Storage, Vol. 1, No. 1, pp. 22–32, Jun. 2023, https://doi.org/10.21595/accus.2022.23077
-
“Intergovernmental panel on climate change.” Geneva, Switz, https://www.ipcc.ch/sr15/
-
W. Musiał, M. Zioło, L. Luty, and K. Musiał, “Energy policy of European union member states in the context of renewable energy sources development,” Energies, Vol. 14, No. 10, p. 2864, May 2021, https://doi.org/10.3390/en14102864
-
S. Malik, P. Makauskas, V. Karaliūtė, M. Pal, and R. Sharma, “Assessing the geological storage potential of CO2 in Baltic Basin: A case study of Lithuanian hydrocarbon and deep saline reservoirs,” International Journal of Greenhouse Gas Control, Vol. 133, p. 104097, Mar. 2024, https://doi.org/10.1016/j.ijggc.2024.104097
About this article
The authors would like to express their sincere gratitude to the Lithuanian Research Council for their generous financial support, which made this research possible, under grant No. P-PD-23-090 and P-PD-22-022. The authors are also deeply appreciative of the Groundwater Laboratory at IIT Roorkee, India, for providing access to their state-of-the-art facilities for conducting the experiments.
The datasets generated during and/or analyzed during the current study are available from the corresponding author on reasonable request.
The authors declare that they have no conflict of interest.