Abstract
Several depleted Cambrian oil fields in Lithuania, with water-cuts reaching 99 %, present promising opportunities for geothermal energy utilization. This study focused on Lithuania’s Cambrian reservoir complex, which has the highest water production rates. The study aimed to explore the advantages of horizontal wells, a technology not previously employed for geothermal production in Lithuania, over traditional vertical wells. To optimize field development, various scenarios were evaluated using mechanistic models, with insights subsequently applied to real field conditions. The results from the mechanistic model demonstrated that horizontal wells outperform vertical wells in both water production and power generation. Furthermore, increasing fracture intensity was shown to enhance water output and power generation by operating the wells at pressures above fracture propagation, facilitating re-injection. However, the study also emphasized the importance of thorough reservoir characterization and modeling to account for geological complexities and improve production outcomes. In conclusion, the research underscores that horizontal wells offer several advantages over vertical wells for geothermal energy production. These include increased water production, higher power generation, reduced drilling costs, and enhanced operational efficiency. These benefits align with technological advancements observed in the hydrocarbon industry, making horizontal wells a viable solution for maximizing geothermal energy potential in Lithuania’s depleted oil and gas fields.
1. Introduction
Lithuania is located on the eastern part of the Baltic sedimentary basin and on the western edge of the Early Precambrian land formation. Lithuania has an unusually high geothermal gradients in its southwestern part, caused by heat-producing elements like thorium, uranium, and potassium within Middle Proterozoic Cratonic granitoid rocks intruding the crystalline basement in several large areas. In western Lithuania, there are three important hydrothermal complexes in the subsurface: one from the Cambrian period (140 m thick), one from the Middle-Lower Devonian period (400 m thick), and one from the Upper-Middle Devonian period (200 m thick) [1].
Lithuania covers an area of around 65,300 km2, with average yearly temperatures ranging from 5 °C to 9 °C, requiring household heating from October through April. To meet this energy requirement, Lithuania’s net energy consumption is 50.8 GJ per capita (14 MWh), suggesting considerable potential for geothermal energy development. Moreover, due to European Union regulation for climate change Lithuania is forced to develop the renewable energy sector and set the goal for Lithuanian Geothermal contribution to 25 % by 2020 which is related to goals to reduce greenhouse gas emissions [1]. Furthermore, despite having a geothermal anomaly in the western part of the country, only one geothermal plant exists in Lithuania, called Klaipeda Geothermal Demonstration Plant (KGDP). Despite initial success, the KGDP ceased operations in 2017 due to operational and financial challenges. The brief history of the geothermal plant can be obtained from the references [1, 2].
2. Purpose and methodology
In Lithuania, the Devonian aquifer is found at a depth of 1135 m, with an average temperature of 45 °C. The rock is loose sandstone, with an average porosity of 26 % and a permeability of 2200 mD. The Cambrian aquifer is deeper, at more than 2000 m, and has temperature ranging between 74 and 96 °C. However, it has a much lower porosity of 7 % and a permeability of 12 mD, making it very tight and hard for water to flow through [1], [3]. To improve geothermal energy production from this tight rock, we need to increase water flow by exposing more of the reservoir and creating artificial fractures. This paper aims to explore whether these methods can successfully boost geothermal energy production by increasing water production rates.
To assess geothermal potential, it has been necessary to develop a geological and simulation model, which represents a key research gap in our study. To better assess the geothermal potential, we created several mechanistic models which were made to represent the important physical processes. After improving the mechanistic models, we will then apply what we learned on a real field site. This will help us evaluate the geothermal potential more accurately and understand how to use this energy source effectively. By doing this, we will connect the gap between theory and real-world use.
The geological screening process involves a detailed examination of the physical properties of certain reservoirs. It looks at factors like porosity, permeability, depth, average underground temperature, water salinity, reservoir pressure, thickness of the reservoir, and Net-to-Gross (NTG) ratio. Additionally, the study assesses the potential for geothermal energy production from Cambrian geothermal complexes in Lithuania, considering geological factors, technical modeling, and the difficulties of converting hydrocarbon wells for geothermal use. Advanced multi-physics models are used to simulate how transport properties change during thermal and hydraulic processes. More details about workflow and applications like mechanistic modeling and uncertainty management like Tornado and Pareto plots can be obtained from the following references [3, 4].
3. Results
As described in the earlier section one geothermal site from Cambrian reservoir has been selected. The detailed properties of the field and power output observed by vertical wells can be obtained from the reference [3] and enhanced workflow including better design of the horizontal wells can be obtained from the reference [4].
Table 1Energy calculations for low, mid, and high cases with fracturing (Mechanistic model which has horizontal well length (2500 m long) with fracturing sensitivity conducted for – 25 years)
Sr. No | Fracture intensity (m) | Case | Average water injection rate (sm3/day) | Average water production rate (sm3/day) | Energy (W) | Energy (MWh) | Energy (GWh) |
1 | 125 | Low Case | 450.76 | 540.62 | 7.725E+14 | 2.14580E+05 | 214.58 |
Mid Case | 492.11 | 603.32 | 8.621E+14 | 2.39466E+05 | 239.47 | ||
High Case | 545.53 | 683.28 | 9.763E+14 | 2.71204E+05 | 271.20 | ||
2 | 250 | Low Case | 313.64 | 399.95 | 5.715E+14 | 1.58746E+05 | 158.75 |
Mid Case | 340.93 | 445.83 | 6.370E+14 | 1.76956E+05 | 176.96 | ||
High Case | 376.63 | 504.67 | 7.211E+14 | 2.00311E+05 | 200.31 | ||
3 | 500 | Low Case | 231.41 | 313.04 | 4.473E+14 | 1.24250E+05 | 124.25 |
Mid Case | 250.79 | 348.77 | 4.984E+14 | 1.38432E+05 | 138.43 | ||
High Case | 276.25 | 394.67 | 5.639E+14 | 1.56650E+05 | 156.65 |
Based on the above methodology we had developed low, mid, and high case models for assessing the geothermal power potential. The results obtained from these models are demonstrated in Fig. 1. The derived energy output from the model is given in Table 1.
Fig. 1Comparison of low, mid, and high case without and with fracturing (mechanistic model)
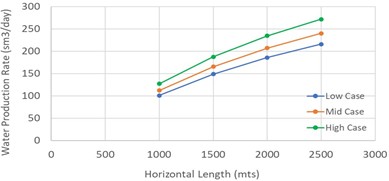
a) Water production rate without fracturing
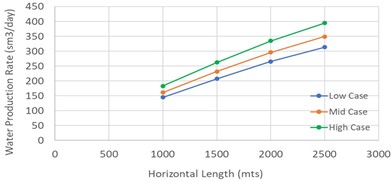
b) Water production rate with fracturing
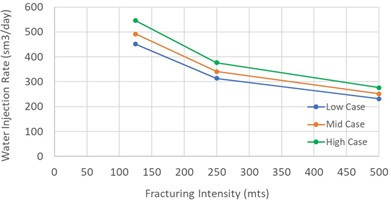
c) Water injection rate with fracturing intensity
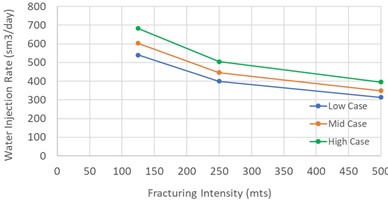
d) Water production rate with fracturing intensity
4. Conclusions
Our analysis shows that the best location for a new horizontal production well in the reservoir depends on factors like the current water temperature, how permeability is spread out, and how close it is to existing wells. Utilizing the mechanistic model, the optimal location for injectors and producers maximizes water production and injection rates with 1200 m well spacing. The study also successfully describes using horizontal wells to enhance water production, injection rates, and power output in geothermal development in Lithuania. The findings show that water production and injection rates increase linearly with horizontal length, while induced fracturing at regular intervals doubles these rates as shown in the mechanistic model. Furthermore, heightened fracture intensity correlates exponentially with increased water production and injection rates, highlighting its significant impact of its application.
Moreover, with the increment in the above parameters, temperature front does not breach the vicinity of the producer well. In mechanistic modeling the study reveals that low, mid, and high cases follow similar water production and reinjection trends, mirroring those in vertical wells.
References
-
A. Memon, P. Makauskas, I. Kaminskaite-Baranauskiene, and M. Pal, “Unlocking geothermal energy: a thorough literature review of Lithuanian geothermal complexes and their production potential,” Energies, Vol. 17, No. 7, p. 1576, Mar. 2024, https://doi.org/10.3390/en17071576
-
A. Rashid, S. Malik, V. Karaliute, P. Makauskas, I. Kaminskaite, and M. Pal, “Lithuania’s geo-energy landscape: a brief overview of CCUS, hydrogen, and geothermal,” Advances in Carbon Capture Utilization and Storage, Vol. 1, No. 2, pp. 33–43, Dec. 2023, https://doi.org/10.21595/accus.2023.23903
-
P. Makauskas, I. Kaminskaite-Baranauskiene, A. Rashid Abdul Nabi Memon, and M. Pal, “Assessing geothermal energy production potential of cambrian geothermal complexes in Lithuania,” Energies, Vol. 17, No. 5, p. 1054, Feb. 2024, https://doi.org/10.3390/en17051054
-
A. R. A. N. Memon, P. Makauskas, I. Kaminskaite-Baranauskiene, and M. Pal, “Workflow for assessing geothermal potential of depleted hydrocarbon fields: a study of Cambrian reservoirs in Lithuania,” ECMOR 2024, No. 1, pp. 1–12, Jan. 2024, https://doi.org/10.3997/2214-4609.202437037
About this article
The authors would like to thank Lithuanian Research Council funding No. “P-MIP-23-102”, Minijos Nafta for their invaluable support in providing the field model for the Vilkyciai reservoir, Innargi and TU Delft for valuable insight and communications.
The datasets generated during and/or analyzed during the current study are available from the corresponding author on reasonable request.
All authors contributed to conceptualization, writing, drafting and editing of the manuscripts.
The authors declare that they have no conflict of interest.