Abstract
The CTS project is evaluating the global potential of direct ship injection technology for facilitating permanent CO2 storage offshore. One of the scenarios analyzed within the project is the Black Sea scenario, exploring potential links and synergies between Romania and Ukraine for implementation of CCUS technology. Romanian scenario will analyze the CCS chain focused on Constanța and Călărași emission clusters, different transport options (including direct ship injection) and offshore storage in deep saline aquifers and hydrocarbon fields from Histria Depression. For Ukraine, the CCS chain includes capture of CO2 from Odesa and Mykolaiv regions, onshore and offshore transport and storage in depleted gas and condensate fields from the Black Sea.
1. Introduction
Traditional solution for offshore storage requires large, costly infrastructure with immense carbon footprint. This hinders the spread of technology especially for smaller emitters and storage operators. The CTS (CO2 Transport and Storage directly from a ship: flexible and cost-effective solutions for European offshore storage) team will investigate how using ships as transport and injection vessels (based on Nemo Maritime AS technology, see Fig. 1) can unlock CCUS potential and speed up its deployment.
CTS will study the impact of direct ship injection on the configuration of capture clusters and storage facilities by developing CCS scenarios in four different offshore regions in Europe: Norwegian Continental Shelf, Baltics, Black Sea and Atlantic coast of Portugal (Fig. 2). The Black Sea scenario is composed from an interlinked Romanian and Ukrainian scenarios.
2. Romanian scenarios
Romanian scenario derives from the one built for implementation of CCUS in Galați region within STRATEGY CCUS project. Due to the industrial decline of the region, lack of interest for CCS and closing of some emitters, new emitters have been considered for the CTS scenario. From the previous project, only offshore storage potential has been kept.
Fig. 1Concept of direct ship injection as presented by NEMO maritime
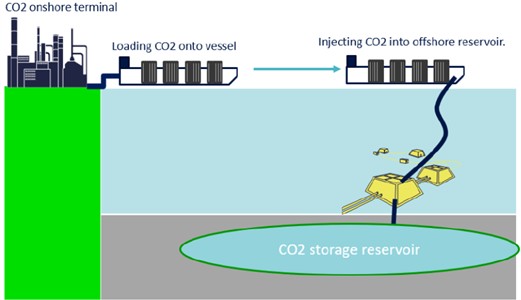
Fig. 2Map of CTS scenarios. Black Sea scenario location is figured with a red circle
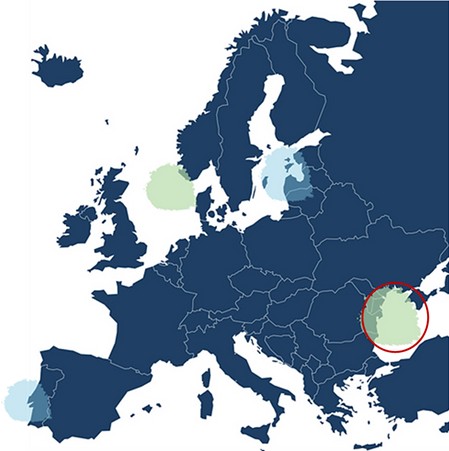
The main Romanian scenario updated for CTS project involves capture of CO2 from two clusters, Călărași and Constanța and storage in the offshore structures (depleted hydrocarbon fields and deep saline aquifers) in the Black Sea (Fig. 3), Romanian exclusive economic zone. For the CCS value chain, a multi-modal transport approach is considered, involving onshore transport to the ports, fluvial transport on the Danube and the Danube-Black Sea channel (upper branch) and maritime transport to the storage sites using three different options, pipeline, conventional shipping and direct ship injection. To facilitate the CO2 transport by ships (fluvial or maritime), three hubs are designed to be located in the main ports, Călărași, Medgidia and Midia.
For Călărași cluster, two emitters are considered, a producer of pig iron and steel and a glass manufacturing facility. These two accounted for 0.15 Mt CO2 eq. in 2023. Constanța cluster has five large emitters, a cement plant from Medgidia, a heat and energy plant in Constanța, an energy plant, a refinery in Midia and a lime factory. These five emitters accounted for 2.03 Mt CO2 eq in 2023. Emitter data, presented in Table 1, were taken from official and public reporting of National Agency for Environmental Protection [1].
Fig. 3Map of Romanian CTS main scenario
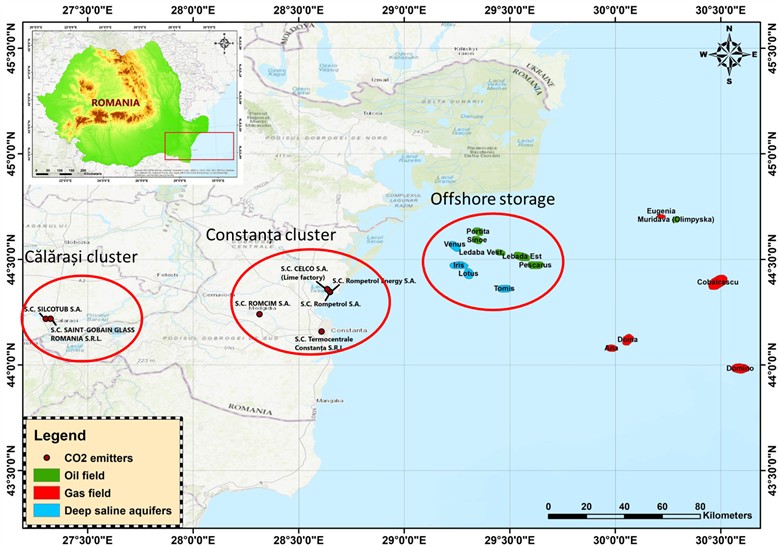
Table 1Emitter data for the two clusters considered under CTS Romanian scenario
Emitter name | Industrial sector | Verified emissions 2023 (t CO2 eq.) | Cluster |
S.C. SILCOTUB S.A. Călărași | Production of pig iron and steel | 48803 | Călărași |
S.C. SAINT – GOBAIN GLASS ROMÂNIA S.R.L. | Glass manufacturing | 100946 | Călărași |
ROMCIM S.A. – Medgidia | Cement production | 853512 | Constanța |
S.C. CELCO S.A. | Lime production | 81793 | Constanța |
S.C. Termocentrale Constanta S.R.L. (former CTE Palas) | Heat and energy production | 110847 | Constanța |
S.C. Rompetrol Rafinare S.A. – Petromidia | Refinery | 835562 | Constanța |
Rompetrol Energy S.A. (former Termoelectric facility Midia S.A.) | Energy production | 150821 | Constanța |
The CO2 captured from emitters listed in Table 1, with approximately 2.18 Mt of CO2 annual emissions, can be stored in offshore storage sites from Black Sea, Romanian exclusive economic zone. One option is represented by deep saline aquifers identified and assessed for CO2 storage in a previous project funded by the Ministry of Research of Romania [2]. These sites, named Venus, Iris, Lotus and Tomis, were selected from the structures found non-productive during the 1980s exploration campaign in the Black Sea. The most suitable storage reservoirs are represented by Eocene carbonates (Venus), quarzitic sandstones with calcareous cement from Albian (Iris) [2], calcareous sandstones with thin intercalations of silty clays within Albian formation (Lotus) [2] and Albian grey sandstones with calcareous cement (Tomis) [2].
The calculation of storage capacity in deep saline aquifers has been made according to the methodology used in EUGeoCapacity project [3]:
where is the storage capacity; NG is net to gross ratio; is the area of the aquifer; is the average thickness of the reservoir; is average reservoir porosity; the density of CO2 at reservoir conditions and is the storage efficiency factor.
It is important to mention that estimates have been made for several of the parameters used, based on the public data, due to the absence of reliable data and considering that access to data related to offshore structures in Romania is very restricted. The estimation of storage capacity is rather conservative, choosing an average porosity of 20 % for all structures, a NG ratio of 0.5 and a storage efficiency of 20 % (Table 2). The total storage capacity calculated for the deep saline aquifers considered, using Eq. (1), reaches 88 Mt of CO2 (Table 2), sufficient for storing the CO2 captured from listed emitters for more than 40 years.
Table 2Estimation of storage capacity for the deep saline aquifers of Romanian scenario
Name | Area (sq km) | Reservoir formation | Depth to top (m) | Average thickness (m) | NG | Porosity (%) | Density of the CO2 | Storage capacity (Mt) |
Iris | 22.1 | Albian | 2600 | 100 | 0.5 | 20 | 650 | 29 |
Venus | 16.55 | Eocene | 1000 | 100 | 0.5 | 20 | 550 | 18 |
Tomis | 17.59 | Albian | 2700 | 144 | 0.5 | 20 | 650 | 33 |
Lotus | 16.05 | Albian | 1523 | 135 | 0.5 | 20 | 650 | 28 |
The second storage option considered is storage in depleted hydrocarbon fields. The selected fields for the Romanian scenario are the oil fields Lebăda Est, Lebăda Vest and Sinoe, exploited for more than 30 years and discovered during the seismic exploration campaign of the Black Sea in the 1980s [4]. Lebăda Est field, discovered in 1980, has three productive reservoirs in Albian (oil), Upper Cretaceous (oil) and Eocene (gas) [4], out of which Albian seems to be the most suited for storage and it is comprised from silicious and calcareous sandstones, microconglomerates and conglomerates with a porosity of 17 % and permeability of 82 mD [5]. Lebăda Vest field, discovered in 1984, has oil reservoirs on Albian and Upper Cretaceous formations and gas in the Eocene formation [4]. The most suitable reservoir for CO2 storage is the Albian, comprised of silicious and calcareous sandstones, microconglomerates and conglomerates, due to its good reservoir properties [5]. Sinoe field, discovered in 1988, has the oil reservoir in Eocene and consists of quartzitic sandstones with clay cement and clay intercalations [5]. For these hydrocarbon fields, estimation of storage capacity has been made only for the oil reservoirs and considering only the most suitable reservoirs. Because of poor data availability, a simple formula was used, based on the EUGeoCapacity project [3]:
where is the storage capacity of the hydrocarbon field; is the CO2 density at reservoir conditions; is the proven ultimate recoverable oil or gas, the sum of cumulative production and the proven reserves and is oil or gas formation volume factor.
Estimated storage capacities are presented in Table 3. Unfortunately, these are only basic estimates since accurate data on ultimate recovery could not be obtained, and publicly available data was used.
Table 3Estimation of storage capacity in Romanian hydrocarbon fields from the Black Sea
Name of the structure | Area (sq km) | Target reservoir (m) | Average porosity (%) | Reservoir depth (m) | Estimated thickness (m) | Oil or gas formation volume factor | Storage capacity oil (Mt) |
Lebăda Est | 21.78 | Albian | 17 | 2300 | 30 | 1.2 | 25 |
Lebăda Vest | 10.13 | Albian | 17 | 2400 | 25 | 1.2 | 25 |
Sinoe | 11.89 | Eocen | 15 | 2000 | 35 | 1.2 | 9 |
The connection between emitters and storage sites is designed in a multi-modal approach presented in Fig. 4. CO2 captured from Călărași emitters can be transported by rail up to an intermittent storage hub to be placed in Călărași commercial port. From this point, the captured CO2 can be transported directly through the Danube and the upper branch of Danube-Black Sea canal into a hub in Midia port. The CO2 captured from Medgidia cement plant can be shipped directly from Medgidia port hub to Midia hub. The CO2 from Termocentrale Constanța can be transported also by rail to Midia hub, as well as the CO2 from the Celco lime factory. The other two emitters, the refinery and the energy plant, are in fact within Midia port.
The suggested value chain scenarios are:
Scenario 1: From Midia hub, CO2 will be transported through an offshore pipeline to a platform from where the CO2 will be distributed to the storage sites. The pipeline is designed to follow the corridor of the existing one that connects the Midia refinery and Gloria platform near Lebăda Est field.
Scenario 2: From Midia hub, CO2 will be loaded on conventional ships and transported to an offshore platform from where the CO2 will be distributed to storage sites.
Scenario 3: From Midia hub, CO2 will be loaded on a ship specific for direct ship injection and injected directly into the storage reservoirs offshore.
Fig. 4Design of CO2 transport for the Romanian scenario
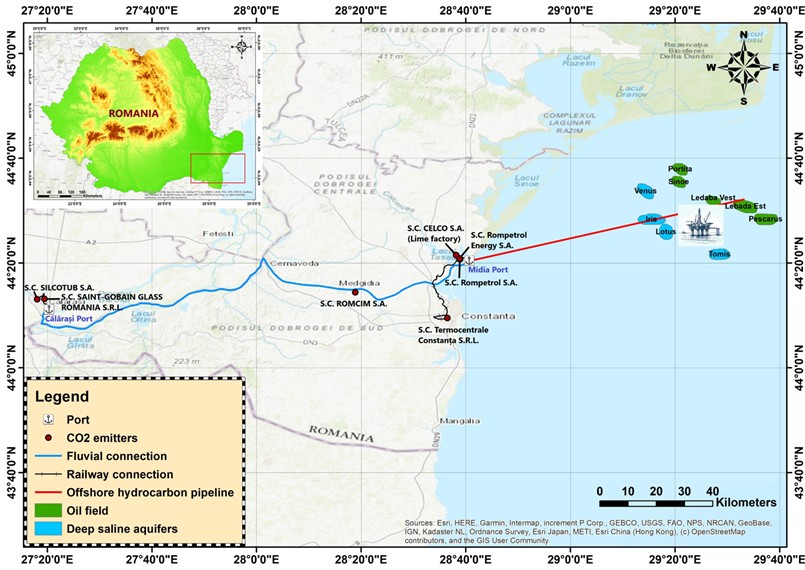
3. Ukrainian CCS scenarios
The Ukrainian base case scenario encompasses both onshore and offshore components. The onshore focus includes the Odesa and Mykolaiv regions with CO2 emissions and key hubs location, while the offshore segment covers the Ukrainian exclusive economic zone in the Black Sea, where potential CO₂ storage sites have been identified (Fig. 5).
Ukrainian scenario is assessed at regional scale and includes 1.26 Mt CO2 emissions from two southern regions - Odesa (Table 4) and Mykolaiv (Table 5), which has significant industrial development and good seaport infrastructure, with reported quantity of CO2 emissions as 0.72 Mt and 0.54 Mt respectively in 2023 [6].
Fig. 5Map with target regions, potential CO2 hubs and storage facilities in Ukraine for CTS project
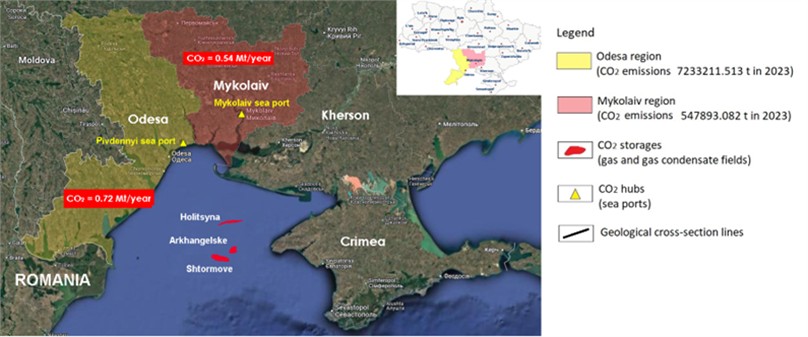
Table 4Quantity of CO2 emissions from stationary sources in Odesa region, Mt. [6]
Year | Number of enterprises, which have CO2 emissions, units | Quantity of CO2 emissions, Mt | Time trend |
2021 | 224 | 1.41 | 89.2 % to 2020 |
2022 | 224 | 0.69* | 49.3 % to 2021 |
2023 | 214 | 0.72* | 104 % to 2022 |
*Data exclude the territories which are temporarily occupied by the Russian Federation and part of territories where the military actions are taking place as of 2024. |
Two other seaside regions (Kherson and Crimea) currently were excluded from analysis due to agricultural focus of economics and significant impact of ongoing war causing doubts in data quality. Western Crimean area can be potential expansion of this study in next research.
Table 5Quantity of CO2 emissions from stationary sources in Mykolaiv region, Mt. [6]
Year | Number of enterprises, which have CO2 emissions, units | Quantity of CO2 emissions, Mt | Time trend |
2021 | 284 | 2.13 | 101.7 % to 2020 |
2022 | 223 | 0.52* | 24.5 % to 2021 |
2023 | 224 | 0.54* | 104.8 % to 2022 |
*Data exclude the territories which are temporarily occupied by the Russian Federation and part of territories where the military actions are taking place as of 2024. |
Pivdennyi and Mykolaiv maritime ports [7], [8] are being considered as strategic CO2 hubs for Odesa and Mykolaiv regions respectively. Their advanced infrastructure includes deep-water berths, high-capacity cranes, conveyors, specialized liquid cargo facilities and extensive storage. Efficient rail and road connections support seamless logistics, reinforcing their role in CO2 transport and storage.
CO2 will be transported to offshore storage sites in the Black Sea, with the geological formations suitable for long-term sequestration. The reservoirs have been identified in depleted gas and gas condensate fields (Holitsyna, Arkhangelske, Shtormove) confined to Karkinite-North Crimean depression. Gas and gas condensate reservoirs, which are considered as potential CO2 storages, primarily referred to Oligocene-Lower Miocene (Maykop series) and Lower Paleocene formations, consist of clay-rich, carbonate (limestones, marls) and terrigenous (sandstones) sediments with porosity ranges from 20 to 30 % at depth from 900 m and up to 2500 m [9]. For these hydrocarbon fields, estimation of storage capacity has been made considering only the most suitable reservoirs. The total preliminary theoretical CO2 storage capacity was estimated at 9.39 Mt using a simple formula from the EUGeoCapacity project (Eq. (2)) (Table 6).
Incomplete subsurface data lead to uncertainty of CO2 storage capacity. Through techno-economic analysis the direct CO2 ship injection into the seabed (NEMO solutions) will be compared with conventional solution of pipelines and ship-based CO2 transport and offshore platforms for injection (Fig. 6).
Table 6Estimation of storage capacity in Ukrainian hydrocarbon fields from the Black Sea [10], [11], [12], [13]
Field and reservoir name | Area, (km2) | Depth (m) | Av. thickness, (m) | Target reservoir | Caprock | MCO2, Mt |
Holitsyna (П-ХІ reservoir) | 43.17 | 2155 | 80 | Lower Paleocene (limestones, marls) and terrigenous (sandstones) sediments) | Clays | 3.33 |
Arkhangelske (M-V reservoir) | 28.6 | 915 | 36 | Maykop (clay and sandy siltstones) | Clays | 1.95 |
Shtormove (П-ХІ reservoir) | 20.25 | 986 | 85 | Lower Paleocene (Microcrystalline fractured limestones) | Clays | 4.11 |
9.39 |
Fig. 6Ukrainian CTS scenarios I-III
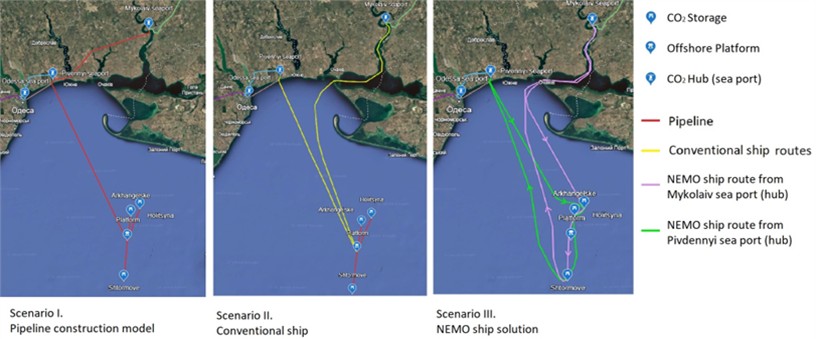
Scenario I: construction of a combined onshore and offshore pipeline system connecting the hubs in the Odesa and Mykolaiv regions to a proposed offshore platform (here and after - Platform) positioned between potential CO2 storage sites. The pipelines, with proposed an estimated total length 294.6 km, will extend from this Platform to each storage, facilitating the transport and injection of CO2.
Scenario II: conventional ships approach to transport CO2 from the hubs to the offshore Platform, with an estimated total routes distance of approximately 670 km. From the Platform CO2 can be transported through pipelines to each of the potential storage sites.
Scenario III: direct ship CO2 injection (NEMO ship solution), with an estimated total routes distance of approximately 820 km.
4. Black Sea scenario
The Black Sea integrated scenario merges the Romanian and Ukrainian scenarios. All emissions are envisioned to be stored primarily in Romanian and potentially also in Ukrainian storage sites. This collaborative model aims to enhance operational efficiency, reduce costs and maximize storage capacity by leveraging shared resources and infrastructure. Key aspects of synergy will include geological complementarity, infrastructure optimization and economic feasibility to determine the most cost-effective and scalable solutions for cross-border CO2 management. The simulations will be used to analyze benefits and potential bottlenecks of cross-border projects, including regulatory aspects. Synergies from cross border cooperation will be estimated in the coming steps and could position the Black Sea region as a strategic hub for CCS in Europe, driving innovation in carbon management and contributing to broader climate goals.
5. Conclusions
In order to check the feasibility of using direct ship injection in the North-Western Black Sea basin several CCS chains were selected for Romania and Ukraine assuming implementation of the technology in comparison with traditional solutions.
For the capture part, only active emitters were taken into consideration and the level of CO2 to be captured was based on 2023 reported and verified emissions. For Romania, two emission clusters were considered, in Călărași and Constanța areas, totalizing 2.18 Mt of CO2. In Ukraine, emissions from two southern regions – Odessa and Mykolaiv, strategically located near the Black Sea, are involved in the selected CCS chain, with a quantity of 0.72 Mt/year, and 0.54 Mt/year respectively. It is worth mentioning that this low level of emissions for the Ukrainian regions is due to the reduction of activities related to the ongoing war. Since there is no guarantee that after the war, the industrial activities and the quantity of emissions will implicitly record a significant increase, for the scenarios to be analyzed within CTS project, 2023 reference values will be taken.
The storage for both scenarios is envisaged offshore in the Black Sea. For Romanian scenario, storage is proposed in deep saline aquifers (Venus, Iris, Tomis, Lotus) and oil fields (Lebăda Est, Lebăda Vest, Sinoe). Holitsyna, Arkhangelske and Shtormove gas and gas condensate fields are considered as potential CO2 storage sites in Ukraine. For both countries and for all the fields, the storage readiness level is low. A conservative estimation on storage capacity has been made for all the fields, based solely on public data, which is extremely limited in Eastern Europe. Due to this fact, several important assumptions had to be made, which could have an impact on the reliability of the estimates. Still, from the estimates it is clear that storage sites can accommodate the captured CO2 for more than 15 years, at the level of 2023 emissions.
The transport component is designed to be multi-modal and involves key hubs in strategic ports, as Călărași, Medgidia and Midia in Romania and Pivdynnyi and Mykolaiv maritime ports in Ukraine. The approach for the transport involves onshore transport (by rail and river for Romania and pipeline for Ukraine) and offshore transport by pipeline, conventional ship and direct ship injection. All these options will be analyzed and compared to assess the feasibility of the technology studied within the CTS project.
After the techno-economic analysis of the designed value chains, representing a simple storage versus emission volumes comparison, an integrated Black Sea scenario will be made, most probably combining storage options. This integration faces though some key risks, related mostly to geological part, regulatory regimes, economic aspects and nonetheless the unfavorable geopolitical framework created by the war. Still, within CTS project, representing an early stage development project, risks are only mapped rather than fully assessed and mitigation actions should be discussed with relevant stakeholders at regional level. Geological risks are in fact associated with poor data availability and include uncertainties about reservoir integrity, injectivity and storage capacity, requiring thorough site-specific assessments and data acquisition campaigns to be conducted at a later stage in the project development. Economically, high infrastructure costs for pipelines, platforms, and ships pose significant challenges, compounded by inflation, supply chain disruptions, and unforeseen technical issues that inflate project budgets. Economic risks will be assessed by evaluating costs of abated CO2 at the scenario level and at individual CCS chain components level and comparing them to ETS (Emission Trading System) predictions. Geopolitical instability, particularly in Ukraine, adds uncertainty to timelines, affects investor confidence, and hinders international collaboration.
Nonetheless, the Black Sea region offers significant potential for scaling up CCS initiatives. This potential can be achieved through the development of large-scale infrastructure and by fostering cross-border cooperation to position Black Sea as a hub for CO2 storage. CCS initiatives should also be integrated with other decarbonization strategies, such as hydrogen production and renewable energy systems. Establishing robust regulatory frameworks, harmonizing cross-border regulations and creating financial incentives will be crucial in attracting investment and ensuring long-term project viability. Additionally, exploring synergies between CCS and emerging technologies, such as coupled carbon storage and geothermal extraction, can enhance project value and mitigate risks. Effective stakeholder engagement will also play a vital role, as collaboration between governments, industry and international partners is necessary to build trust and secure lasting commitments for the achievement of regional decarbonization goals. With proper risk mitigation, meticulous planning and a forward-looking approach, the Black Sea region has the potential to become a leader in sustainable and scalable CCS solutions, contributing to worldwide decarbonization efforts.
References
-
“The emissions trading scheme for greenhouse gases/Verified emissions 2021-2030.” ANPM. https://www.anpm.ro/schema-de-comercializare-a-emisiilor-de-gaze-cu-efect-de-sera/-/asset_publisher/8rwxognz1xru/content/emisii-verificate (accessed 2024).
-
A. Dudu, I. Morosanu, C. Sava, G. Iordache, C. Avram, and S. Anghel, “CO2 geological storage possibilities in Histria Depression – Black Sea,” GeoEcoMarina, Vol. 23, pp. 171–176, Dec. 2017.
-
T. Vangkilde-Pedersen et al., “Assessing European capacity for geological storage of carbon dioxide-the EU GeoCapacity project,” Energy Procedia, Vol. 1, No. 1, pp. 2663–2670, Feb. 2009, https://doi.org/10.1016/j.egypro.2009.02.034
-
Moroșanu and I., “The hydrocarbon potential of the Black Sea continental Plateau in Romania,” Romanian Journal of Earth Sciences, Vol. 86, No. 2, pp. 91–109, 2012.
-
D. Luțac, “Sedimentary and tectonic processes at the base of the paleoslope of the Histria Basin,” (in Romanian), University of Bucharest, Faculty of Geology and Geophysics, Bucharest, 2000.
-
“State Statistics Service of Ukraine,” https://www.ukrstat.gov.ua/
-
“Mykolaiv sea port,” https://mmtp.com.ua/
-
“Pivdennyi sea port,” https://www.port-yuzhny.com.ua/
-
V. Mykhailov et al., “Unconventional sources of hydrocarbons of Ukraine: monograph. In eight books. Southeastern oil and gas bearing region,” (in Ukrainian) in Analytical Investigations, Vol. 3, 2014.
-
D. E. Makarenko, “Arkhangelske gas field,” in Encyclopedia of Modern Ukraine, 2001.
-
D. E. Makarenko, “Holitsyna gas condensate field,” in Encyclopedia of Modern Ukraine, 2006.
-
M. Pavlyuk and M. Yakovenko, “Oil and gas bearing potential sea areas of the East European Platform,” Geology and minerals of the World Ocean, No. 1, pp. 32–46, 2019.
-
R. Kondrat, M. Kharitonov, O. Kondrat, and P. Melnychuk, “Features of the development and operation of the Arkhangelske gas field and ways of increase efficiency gas production and gas extraction coefficient,” Exploration and Development of Oil and Gas Fields, No. 2, pp. 66–69, 2006.
About this article
This research was funded by CETPartnership, the Clean Energy Transition Partnership under the 2022 CETPartnership joint call for research proposals, co-funded by the European Commission (GA N. 101069750) and with the funding organizations detailed on https://cetpartnership.eu/funding-agencies-and-call-modules.
The datasets generated during and/or analyzed during the current study are available from the corresponding author on reasonable request.
Alexandra-Constanța Dudu: writing-original draft preparation, conceptualization. Yuliia Demchuk: writing, review and editing, conceptualization. Ivan Virshylo, Mariia Kurylo, Roman Berenblyum, Anders Nermoen, Gabriel Iordache, Andrei-Gabriel Dragoș, Constantin-Ștefan Sava, Corina Avram, Lia Stelea, Mykhailo Bratakh, Leonid Melnyk: conceptualization.
The authors declare that they have no conflict of interest.