Abstract
This paper establishes a quantitative design method for the durability of concrete structures in cross-sea bridges through investigation, rapid chloride migration coefficient method (RCM) and theoretical calculation, considering the impact of temperature on chloride ion diffusion rates in a hot marine salt erosion environment. Combined with the RCM test and bridge service data, a quantitative design method for bridge concrete durability is proposed. Test results show that the growth rate of the chloride ion diffusion coefficient of concrete is approximately 1.028 for every 1 °C increase. For every 5 °C increase, the growth rate of the chloride diffusion coefficient of concrete is about 1.15, and the cover depth of the concrete structure should be multiplied by a coefficient of 1.07. Therefore, the concrete cover depth should be appropriately increased, considering the influence of ambient temperature. Furthermore, fly ash, slag, and stone powder can increase the concrete’s resistance to chloride corrosion. When the influence of temperature on the chloride ion diffusion coefficient is considered, the durability design of the concrete structure of the sea-crossing bridge is conducted, which is beneficial for ensuring their service life.
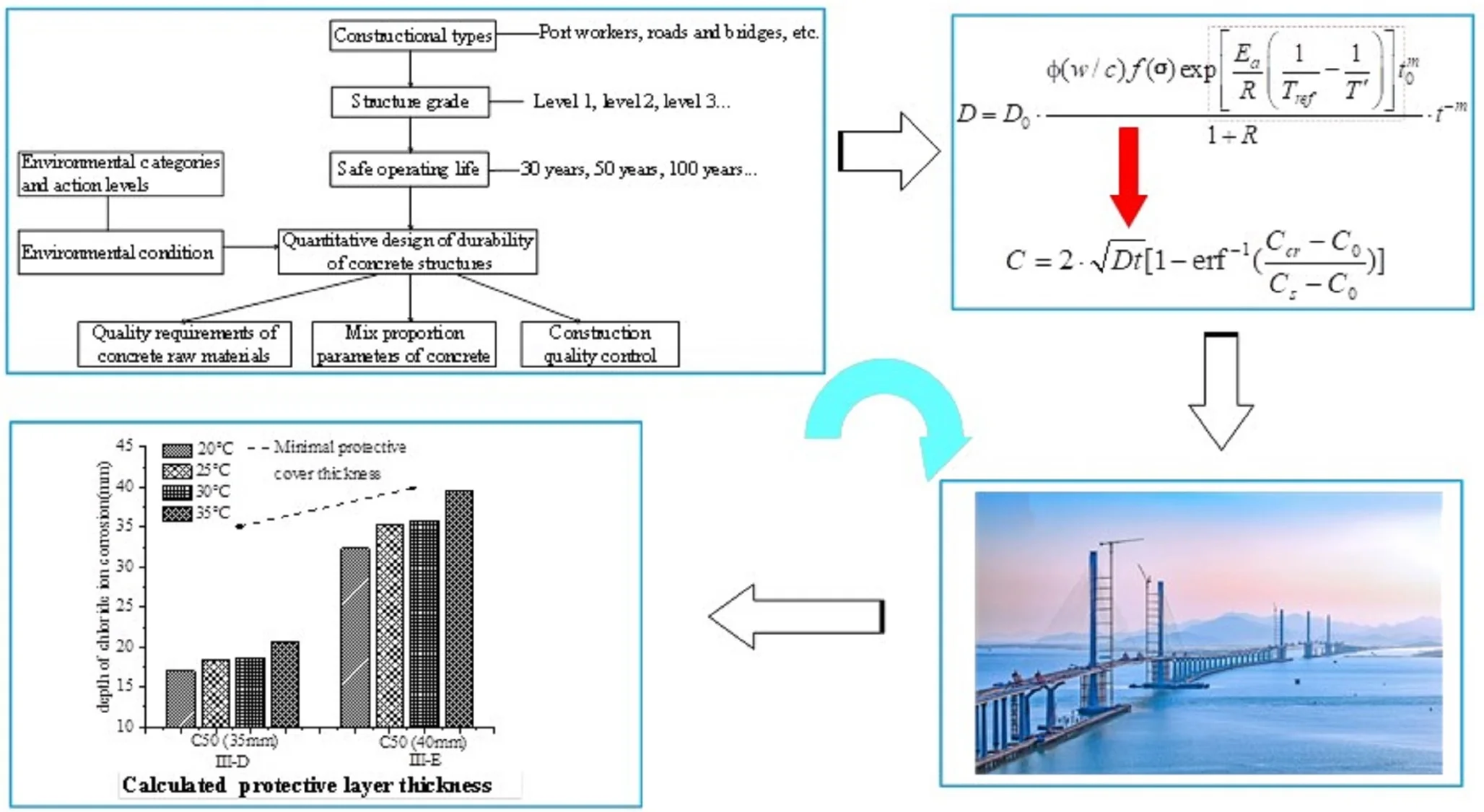
Highlights
- This paper establishes a quantitative design method for the durability of concrete structures in cross-sea bridges.
- Combined with the RCM test and bridge service data, a quantitative design method for bridge concrete durability is proposed.
- The concrete cover depth should be appropriately increased, considering the influence of ambient temperature.
1. Introduction
Numerous factors influence the durability of concrete structures in marine environments, including environmental temperature, humidity, exposure position, and the concrete age [1-6]. According to the Code for Durability Design of Concrete Structures in Highway Engineering (JTG/T3310-2019), it is a hot area where the annual average temperature exceeds 20 °C. The “Guangdong-Hong Kong-Macao Greater Bay Area Climate Monitoring Bulletin” [7], jointly issued by the meteorological departments of Guangdong, Hong Kong, and Macao indicates that the average temperature has been above 21 °C from 1961 to 2021 in the Greater Bay Area, with an annual increase of 0.03 °C. The average temperature in 2021 was 23.5 °C; in 2022, it was 22.7 °C. The temperature has remained above 22.5 °C in recent years, as shown Fig. 1.
It is crucial research focusing on the durability design of reinforced concrete structures [8-13]. The basic concept for designing the durability of concrete structures in a marine salt erosion environment is as follows: first, the service life of concrete structures based on building category and grade should be determined, then the concrete raw materials [14], concrete mix ratio parameters, and quality control of concrete construction can be established by the quantitative durability design of concrete structures [15-22]. The quantitative design aims to ensure concrete structure durability by adjusting parameters such as water-binder ratio, concrete strength, and protective layer thickness [23-27] (see Fig. 2).
Fig. 1The annual variation of average temperature in the Greater Bay Area from 1961 to 2021
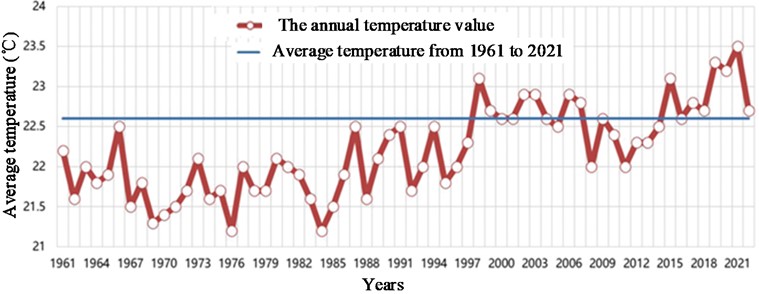
Fig. 2Quantitative design diagram for the durability of reinforced concrete structures
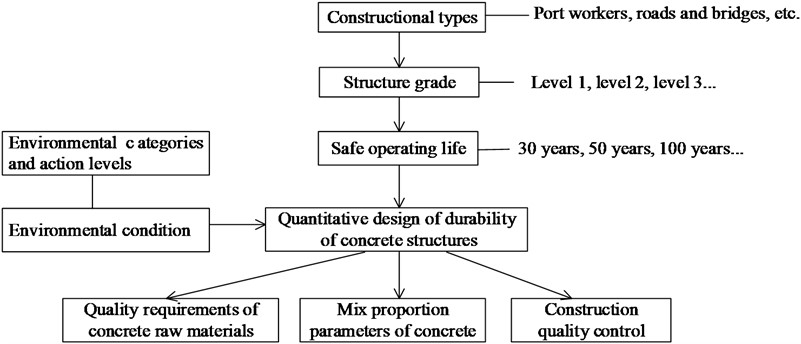
The design of marine concrete structures' durability relies primarily on engineering expertise and qualitative analysis [28, 29]. Hence, ensuring the designed service life for concrete structures poses a significant challenge. The commonly used Fick’s second law chloride ion erosion model mainly considers two boundary conditions: the initial chloride ion concentration inside the concrete structure and the chloride ion concentration on the surface. In accordance with the “Code for Durability Design of Concrete Structures for Highway Engineering” (JTG/T 3310-2019), the environmental category is classified as the offshore or marine chloride environment. The determination is predominantly categorized into moderate, severe, very critical, and extremely severe environmental effects, based on the investigation of the annual average temperature, the hottest and coldest monthly average temperatures, the distance from the coastline, and the position of the component in the seawater environment [30-34]. However, attaining this proves highly challenging due to the lack of quantitative environmental effect indicators and the dearth of a quantitative nexus between environmental effects and concrete durability indicators [35, 36].
Based on an analysis of the degradation mechanism of chloride corrosion in concrete, this paper quantitatively assesses the influence of temperature on chloride corrosion. It takes temperature, relative humidity, and chloride ion concentration as control parameters to establish a quantitative index of the chloride corrosion environment. Then, the theoretical model of chloride ion erosion, grounded on Fick’s second law, is modified by introducing correction coefficients for temperature, relative humidity, chloride ion concentration, and other factors. Subsequently, a practical prediction model for the analysis of chloride ion erosion of concrete is constructed. The service life and durability design parameters of concrete structures in hot marine salt erosion environments are quantitatively analyzed, and the validity and applicability of the method are verified by comparing the experimental data.
2. Materials and methods
2.1. Raw materials and mix proportion
The concrete strength grades are C30, C40, and C50, corresponding design water-binder ratios of 0.38, 0.36, and 0.31. The cement used is PII42.5 Portland cement produced by Yingde Conch Cement Co., Ltd. The chemical composition of the cement, slag, and fly ash is presented in Table 1. After testing, the indicators meet the product standards. Natural river sand is used as the fine aggregate, while natural gravel with a particle size gradation of 5-25 mm and a maximum particle size of 25mm is used as the coarse aggregate. Laboratory tap water is used as the mixing water. The concrete mix design is shown in Table 2.
Table 1Chemical composition of cement (%)
Chemical composition | SO3 | SiO2 | Al2O3 | Fe2O3 | Cao | MgO | Na2O | K2O |
P∙II42.5 | 2.9 | 22.11 | 5.12 | 4.23 | 64.11 | 0.87 | 0.45 | 0.21 |
Slag | 0.89 | 31 | 14.2 | 2.08 | 40.95 | 7.75 | – | – |
Fly ash | 0.83 | 51.04 | 32.86 | 8.26 | 3.35 | – | 0.36 | 0.5 |
Table 2Concrete mix proportion (kg/m3)
No. | Water-binder ratio | Cement | Slag | Fly ash | Stone powder | Sand | Gravel | Water |
A | 0.31 | 350 | 130 | 0 | 0 | 702 | 1248 | 150 |
B | 0.36 | 280 | 140 | 0 | 14.28 | 714 | 1116 | 156 |
C | 0.38 | 155 | 90 | 120 | 0 | 764 | 1246 | 140 |
2.2. Test methods
The concrete was poured into moulds of Φ100 mm×50 mm. The specimens underwent film curing for 24 hours before moulding and were then removed after an additional 24 hours. Curing occurred in a standard room at 20 °C±2 °C and a relative humidity of 95 %. After 28 days, testing was conducted following GB/T 50082-2009, “Standard Test Method for Long-term Performance and Durability of Ordinary Concrete.” The chloride ion diffusion coefficient of concrete was tested under different temperature conditions (20 ℃, 25 °C, and 30 °C), and the thickness of the concrete protective layer was determined.
Considering the impact of temperature on the chloride diffusion coefficient [12], the calculation equation is as follows:
where and are the chloride diffusion coefficient at 20 °C and , respectively; is the apparent activation energy, and it can be taken as 20 kJ/mol in the absence of experimental data; is the perfect gas constant, 8.31×10-3kJ/(mol∙K-1).
Besides examining the impact of temperature on the chloride ion diffusion coefficient, we also need to assess the effects of various factors, including cement composition, curing age, load, and water-cement ratio, on the diffusion coefficient of chloride ions [12]:
where is the influence coefficient of water-cement ratio on chloride ion diffusion coefficient of concrete. is the influence coefficient of load on chloride diffusion coefficient of concrete.
According to JT/T 985-2015, the durability requirements for concrete used in highway bridges and culverts specify that the chloride diffusion coefficient should not fall below the values listed in Table 3. By referencing the chloride ion diffusion coefficient [12] in Table 3, the chloride ion diffusion coefficient at various temperatures can be calculated using Eq. (2). For instance, if the average annual temperature is 22 °C and the maximum temperature in July and August exceeds 35 °C, it becomes essential to account for the effect of temperature on the durability of concrete structures.
Table 3The requirements of the chloride diffusion coefficient
Grades | III-C, III-D | III-E | III-F | |||
Years | 100 | 50 or 30 | 100 | 50 or 30 | 100 | 50 or 30 |
×10-12 m2/s | 8 | 10 | 5 | 7 | 4 | 5 |
The concentration of chloride ions in concrete varies with time and thickness of the concrete. We have established the relationship between the concentration of chloride ions, time, and position. The distribution of chloride ion concentration can be expressed as:
where is the initial concentration of chloride ion in the concrete, is the chloride ion concentration on exposed surface of concrete, erf is the error function, .
According to the concentration distribution of chloride ion in concrete, the thickness of concrete eroded by chloride ion can be calculated:
When the chloride ion concentration on the surface of the steel bar in the concrete reaches the chloride threshold value, the thickness of the protective layer of the concrete structure can be expressed as:
3. Results and discussion
3.1. The effect of temperature on the diffusion coefficient
The chloride ion diffusion coefficient of concrete with different cementitious materials at different ambient temperatures is shown in Table 4 and Fig. 3. When the water-binder ratio is 0.31, the chloride diffusion coefficient at 20 °C, 25 °C and 30 °C are 1.25×10-12 m2/s, 1.48×10-12 m2/s, and 1.52×10-12 m2/s, respectively. When the water-binder ratio is 0.36, the chloride diffusion coefficient at 20 °C, 25 °C and 30 °C are 1.34×10-12 m2/s, 1.63×10-12 m2/s, and 1.84×10-12 m2/s, respectively. When the water-binder ratio is 0.38, the chloride diffusion coefficient at 20 °C, 25 °C and 30 °C are 1.93×10-12 m2/s, 2.25×10-12 m2/s, and 2.7×10-12 m2/s, respectively. With the increase of temperature, the chloride ion diffusion coefficient increases linearly. The calculated chloride diffusion coefficient is consistent with the experimental results, and the growth slope can be expressed as:
When the apparent activation energy and gas constant of concrete are 20 kJ/mol and 8.314 kJ/(mol∙K-1) respectively, the growth rate of chloride ion diffusion coefficient of concrete is about 1.028 for every 1 °C increase. For every 5 °C increase, the growth rate of chloride diffusion coefficient of concrete is about 1.15, which can be easily used to calculate the thickness of protective layer of concrete.
Table 4The effect of temperature on the chloride diffusion coefficient.
Item | The chloride diffusion coefficient / (×10-12 m2/s) | ||
20 °C | 25 °C | 30 °C | |
A | 1.25 | 1.48 | 1.52 |
B | 1.34 | 1.63 | 1.84 |
C | 1.93 | 2.25 | 2.70 |
Fig. 3The chloride diffusion coefficient at different temperatures
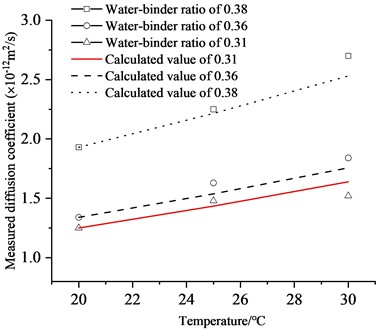
3.2. The effect of water-binder ratio on the diffusion coefficient
In this paper, three water-binder ratios of 0.31, 0.0.36 and 0.38 are studied. Fig. 4 shows the relationship between the diffusion coefficient and the water-binder ratio. Under the given chloride ion concentration and temperature, concrete's chloride ion diffusion coefficient increases with the increase of the water-binder ratio. If the diffusion coefficient is the ordinate, the water-binder ratio is the abscissa. The water-binder ratio significantly affects the concrete with fly ash and stone powder, and the concrete with slag has little influence on the diffusion coefficient. The hydration characteristics of various gelling materials can explain this difference in the analysis below.
3.3. The effect of mineral admixture on the diffusion coefficient
The researchers believe that slag and fly ash can improve the impermeability of concrete. From the test data of this paper, it can be analyzed that slag and fly ash can reduce the chloride ion permeability of concrete, which is a long-term process. In the test, the concrete specimens were only cured for 28 days. The chloride ion erosion test of concrete was carried out, and fly ash, slag and stone powder were not involved in the hydration process. The test data shows that adding 32.9 % fly ash when the water-binder ratio is 0.38 does not significantly differ from the test results at 20 °C for water-binder ratios of 0.36 and 0.38, indicating that the addition of fly ash benefits the resistance to chloride ion penetration of concrete. The water-binder ratio’s ability of resist concrete chloride corrosion is greater than that of mineral admixtures. In Reference [37], the chloride diffusion coefficients of ordinary concrete, 20 % fly ash concrete, 40 % slag concrete and 5 % silica fume concrete were tested according to NT build 443 and NT build 492, respectively. The results show that the addition of fly ash to high water-binder ratio concrete is not conducive to improving impermeability, while the addition of fly ash to low water-binder ratio concrete can improve its impermeability, as shown in table 5. Both 40 % slag and 5 % silica fume can improve the impermeability of concrete. In reference [38], the chloride diffusion coefficient of concrete at 22 °C, 35 °C and 50 °C with a water-binder ratio of 0.4 and silica fume content of 5 % tested. Compared with ordinary concrete, the chloride diffusion coefficient decreased by 52 %, 50 % and 51 %, respectively.
Fig. 4Chloride diffusion coefficient under different water-binder ratio
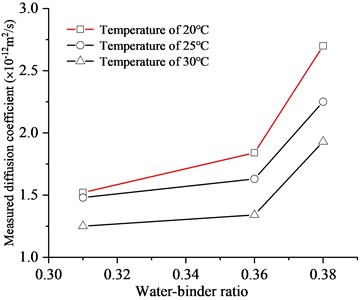
Table 5Influence of mineral admixture on the diffusion coefficient [37]
Concrete type | NT build 443 | NT build 492 | ||||
0.6 | 0.48 | 0.35 | 0.6 | 0.48 | 0.35 | |
Blank concrete | 1 | 1 | 1 | 1 | 1 | 1 |
20 % Fly ash concrete | 115.5 % | 156.4 % | 40.2 % | 113.7 % | 94.1 % | 60 % |
40 % Slag concrete | 36.3% | 108.8 % | 38.7 % | 38.1 % | 86.8 | 28.7 % |
5 % Silica fume concrete | 42.4 | 86.8 % | 38.1 % | 28.7 % | 48.4 % | 16.1 % |
Based on the results of this study, different types of mineral admixtures and water-binder ratios have different effects on the resistance of concrete to chloride corrosion. Therefore, when the thickness of the concrete protective layer is designed, it is necessary to select the appropriate water-binder ratio and the type and content of mineral admixtures. In addition, good construction technology is also a critical factor in ensuring the crack resistance and durability of concrete.
3.4. Example analysis and calculation
The Gaolangang Bridge of the Huangmaohai Sea Cross-sea Channel Project is situated in a hot marine environment, specifically in a marine chloride environment. The calculation parameters are presented in Table 6. The design service life is 100 years. With a water-binder ratio of 0.31, the chloride ion diffusion coefficient is 1.25×10-12 m2/s at 20 °C, 1.48×10-12 m2/s at 25 °C, and 1.52×10-12 m2/s at 30 °C. The chloride ion diffusion coefficient at 35 °C is calculated to be 1.86×10-12 m2/s according to Eq. (1). In accordance with the “Code for Durability Design of Concrete Structures in Highway Engineering” (JTG/T3310-2019), the chloride ion concentration and critical chloride ion concentration on the concrete structure’s surface were used to calculate the protective layer thickness of the box girder. The calculated thickness of the protective layer, considering the influence of 25 °C or the maximum temperature of 30 °C, is presented in Table 7 and Fig. 5.
At 20 °C, the chloride ion erosion depth under different environmental action levels is less than the minimum protective layer thickness specified in the ‘Code for Durability of Concrete Structures for Highway Engineering’ (JTG T3310-2019). This suggests that when the box girder reaches its 100-year design service life, chloride ions do not erode the surface of the stressed steel bar, thus preventing steel bar corrosion. At environmental action level III-E, with a maximum temperature of 35 °C, the calculated chloride ion erosion depth is 39.5 cm, which is only 0.5 mm different from the design value of the concrete protective layer thickness of 40 mm. This suggests that the specified minimum protective layer thickness no longer meets the durability design requirements due to temperature effects. Thus, an increase in protective layer thickness or the implementation of additional anti-corrosion measures is required.
Table 6Parameters of concrete box girder and environmental conditions
Essential parameter | Parameter value | ||||
Environmental parameter | Average temperature | 20 ℃ | 25 ℃ | 30 ℃ | 35 ℃ |
Environmental category | Offshore or marine chloride environment | ||||
Environmental grade | Ⅲ-D | ||||
Ⅲ-E | |||||
Component performance parameters | Concrete grades | C50 | |||
The water-binder ratio | 0.31 | ||||
Design service life (years) | 100 |
Table 7The chloride ion erosion depth and minimum protective layer thickness in the specification
Environmental grade | Minimum protective layer thickness (mm) | (kg/m3) | (kg/m3) | The chloride ion erosion depth (mm) | |||
20 ℃ | 25 ℃ | 30 ℃ | 35 ℃ | ||||
Ⅲ-D | 35 | 1.5 | 2.400 | 17.0 | 18.5 | 18.7 | 20.7 |
Ⅲ-E | 40 | 1.5 | 4.704 | 32.3 | 35.2 | 35.7 | 39.5 |
Fig. 5Compared with the calculated chloride ion erosion depth and the minimum protective layer thickness in the specification
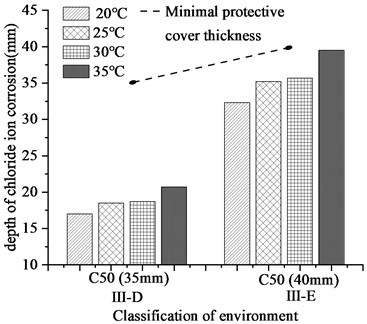
The design of the protective layer thickness of concrete follows Eq. (5). The environmental action level remains constant, meaning that the chloride ion concentration on the concrete surface equals the critical chloride ion concentration. The chloride ion diffusion coefficient of concrete at a room temperature of 20 °C is already known. Considering the influence of ambient temperature on the chloride ion diffusion coefficient, the growth rate of the chloride ion diffusion coefficient can be calculated by Eq. (6). For every 5 °C increase, the growth rate of the chloride ion diffusion coefficient is 1.15. The protective layer thickness of the concrete structure should be multiplied by a coefficient of 1.07. When the temperature does not increase by 5 °C, the reduction coefficient can be calculated through linear interpolation.
4. Conclusions
This paper establishes a quantitative design method for the durability of bridge concrete based on a deterioration mechanism model in a hot marine salt erosion environment, drawing from the durability design methods. The following conclusions have been drawn:
1) The average temperature in recent years has been above 22.5 °C, and the temperature in the vicinity exhibits a gradual upward trend over time, which is detrimental to the durability of concrete structures. The impact of high ambient temperature on the durability of concrete in sea-crossing bridges should be considered.
2) The test results show that the chloride diffusion coefficient rises linearly with the temperature increasing from 20 °C to 30 °C. For every 5 °C rise, the growth rate of the chloride ion diffusion coefficient is 1.15. In cases where the temperature does not increase by 5 °C, the reduction coefficient can be calculated using linear interpolation.
3) Through analysis and calculation, this study shows that the thickness of the concrete protective layer should be multiplied by a coefficient of 1.07 for every 5 °C increase in ambient temperature.
4) Considering the quantitative design of the durability of concrete structures under hot marine salt erosion, the durability design method may comprehensively include increasing the thickness of the protective layer or implementing additional anti-corrosion measures to enhance the service life of bridge concrete structural members.
References
-
Z. Zhuang, S. Mu, Z. Guo, G. Liu, J. Zhang, and C. Miao, “Diffusion-reaction models for concrete exposed to chloride-sulfate attack based on porosity and water saturation,” Cement and Concrete Composites, Vol. 146, p. 105378, Feb. 2024, https://doi.org/10.1016/j.cemconcomp.2023.105378
-
J. Park et al., “Monitoring long-term chloride penetration into wave power marine concrete structures under repeated air pressure,” Construction and Building Materials, Vol. 428, p. 136276, May 2024, https://doi.org/10.1016/j.conbuildmat.2024.136276
-
J.-J. Chen, R.-J. Wu, K. Chen, Z. Wang, and J. Xia, “Influence of initial saturation degree on chloride transport in concrete under hydraulic pressure,” Journal of Building Engineering, Vol. 87, p. 108897, Jun. 2024, https://doi.org/10.1016/j.jobe.2024.108897
-
J. Liu, C. Liao, H. Jin, Z. Jiang, and H. Zhou, “Numerical and experimental research on the effect of rainfall on the transporting behavior of chloride ions in concrete,” Construction and Building Materials, Vol. 302, p. 124160, Oct. 2021, https://doi.org/10.1016/j.conbuildmat.2021.124160
-
J. Bao, R. Zheng, J. Wei, P. Zhang, S. Xue, and Z. Liu, “Numerical and experimental investigation of coupled capillary suction and chloride penetration in unsaturated concrete under cyclic drying-wetting condition,” Journal of Building Engineering, Vol. 51, p. 104273, Jul. 2022, https://doi.org/10.1016/j.jobe.2022.104273
-
L. Liu, S. Zhao, J. Xin, and Z. Wang, “Simplified analysis of thermal cracks in low‐heat Portland cement concrete,” Advances in Civil Engineering, Vol. 2022, No. 1, p. 76305, Apr. 2022, https://doi.org/10.1155/2022/7630568
-
W. Liu, R. Hu, J. Zheng, Z. Li, T. Tang, and Z. Tan, “Guangdong-Hong Kong-Macao greater bay area climate bulletin,” (in Chinese), Guangdong Meteorological Bureau, Hong Kong Observatory and Macao Geophysics and Meteorology Bureau, 2023.
-
F. Bahman-Zadeh, A. Zolfagharnasab, M. Pourebrahimi, M. Mirabrishami, and A. A. Ramezanianpour, “Thermodynamic and experimental study on chloride binding of limestone containing concrete in sulfate-chloride solution,” Journal of Building Engineering, Vol. 66, p. 105940, May 2023, https://doi.org/10.1016/j.jobe.2023.105940
-
C. Jiang, C. Song, X.-L. Gu, Q. Zhang, and W.-P. Zhang, “Modeling electrochemical chloride extraction process in cement-based materials considering coupled multi-ion transports and thermodynamic equilibriums,” Journal of Cleaner Production, Vol. 401, p. 136778, May 2023, https://doi.org/10.1016/j.jclepro.2023.136778
-
Q.-F. Liu et al., “Numerical study of interactive ingress of calcium leaching, chloride transport and multi-ions coupling in concrete,” Cement and Concrete Research, Vol. 165, p. 107072, Mar. 2023, https://doi.org/10.1016/j.cemconres.2022.107072
-
O. O. Mssi et al., “Understanding the degradation mechanisms of cement-based systems in combined chloride-sulfate attack,” Cement and Concrete Research, Vol. 164, p. 107065, Feb. 2023, https://doi.org/10.1016/j.cemconres.2022.107065
-
Q. Li, Z. Chen, L. Liu, S. Zhao, and S. Wang, “Study on durability of concrete bridge against chloride lon erosion in hot marine environment,” (in Chinese), Journal of Highway and Transportation Research and Development, Vol. 40, No. 5, pp. 72–77, May 2023, https://doi.org/10.3969/j.issn.1002-0268.2023.05.010
-
R. Douglas Hooton, “Future directions for design, specification, testing, and construction of durable concrete structures,” Cement and Concrete Research, Vol. 124, p. 105827, Oct. 2019, https://doi.org/10.1016/j.cemconres.2019.105827
-
M. I. A. Othman and I. A. Abbas, “Effect of rotation on plane waves at the free surface of a fibre-reinforced thermoelastic half-space using the finite element method,” Meccanica, Vol. 46, No. 2, pp. 413–421, Aug. 2010, https://doi.org/10.1007/s11012-010-9322-z
-
Kefei Li, Huizhen Lian, and Xiaotan Di., “Durability design of concrete structures: principle, method and standard,” (in Chinese), China Civil Engineering Journal, Vol. 54, No. 10, pp. 64–71, 2021, https://doi.org/10.15951/j.tmgcxb.2021.10.010
-
R. Gupta, B. Lukić, A. Tengattini, F. Dufour, and M. Briffaut, “Experimental characterisation of transient condensed water vapour migration through cracked concrete as revealed by neutron and x-ray imaging: Effect of initial saturation,” Cement and Concrete Research, Vol. 162, p. 106987, Dec. 2022, https://doi.org/10.1016/j.cemconres.2022.106987
-
O. H. A. Dehwah and Y. Xi, “Theoretical model for the coupling effect of moisture transport on chloride penetration in concrete,” Cement and Concrete Research, Vol. 177, p. 107431, Mar. 2024, https://doi.org/10.1016/j.cemconres.2024.107431
-
J. Bao, J. Wei, P. Zhang, Z. Zhuang, and T. Zhao, “Experimental and theoretical investigation of chloride ingress into concrete exposed to real marine environment,” Cement and Concrete Composites, Vol. 130, p. 104511, Jul. 2022, https://doi.org/10.1016/j.cemconcomp.2022.104511
-
I. A. Abbas and M. I. Othman, “Generalized thermoelastic interaction in a fiber-reinforced anisotropic half-space under hydrostatic initial stress,” Journal of Vibration and Control, Vol. 18, No. 2, pp. 175–182, Jun. 2011, https://doi.org/10.1177/1077546311402529
-
I. A. Abbas, “A two-dimensional problem for a fibre-reinforced anisotropic thermoelastic half-space with energy dissipation,” Sadhana, Vol. 36, No. 3, pp. 411–423, Jul. 2011, https://doi.org/10.1007/s12046-011-0025-5
-
I. A. Abbas, “A GN model for thermoelastic interaction in an unbounded fiber-reinforced anisotropic medium with a circular hole,” Applied Mathematics Letters, Vol. 26, No. 2, pp. 232–239, Feb. 2013, https://doi.org/10.1016/j.aml.2012.09.001
-
I. A. Abbas and A. M. Zenkour, “The effect of rotation and initial stress on thermal shock problem for a fiber-reinforced anisotropic half-space using Green-Naghdi theory,” Journal of Computational and Theoretical Nanoscience, Vol. 11, No. 2, pp. 331–338, Feb. 2014, https://doi.org/10.1166/jctn.2014.3356
-
Jiande Xiu, Zuquan Jin, Ning Li, and Baorong Hou., “research progress of chloride ion transport in concrete under marine salt spray environment,” Bulletin of the Chinese Ceramic Society, Vol. 42, No. 3, pp. 771–785, 2023, https://doi.org/10.16552/j.cnki.issn1001-1625.20230206.003
-
H. Jin et al., “An experimental study on the influence of continuous ambient humidity conditions on relative humidity changes, chloride diffusion and microstructure in concrete,” Journal of Building Engineering, Vol. 59, p. 105112, Nov. 2022, https://doi.org/10.1016/j.jobe.2022.105112
-
I. A. Abbas, “Fractional order GN model on thermoelastic interaction in an infinite fibre-reinforced anisotropic plate containing a circular hole,” Journal of Computational and Theoretical Nanoscience, Vol. 11, No. 2, pp. 380–384, Feb. 2014, https://doi.org/10.1166/jctn.2014.3363
-
A. M. Zenkour and I. A. Abbas, “Thermal shock problem for a fiber-reinforced anisotropic half-space placed in a magnetic field via G-N model,” Applied Mathematics and Computation, Vol. 246, pp. 482–490, Nov. 2014, https://doi.org/10.1016/j.amc.2014.08.052
-
I. A. Abbas, “A dual phase lag model on thermoelastic interaction in an infinite fiber-reinforced anisotropic medium with a circular hole,” Mechanics Based Design of Structures and Machines, Vol. 43, No. 4, pp. 501–513, Oct. 2015, https://doi.org/10.1080/15397734.2015.1029589
-
J. Yue, Z. Shen, L. Xu, and K. Zhang, “Numerical analysis the calcium leaching effect in concrete and concrete-rock interface on concrete gravity dam anti-sliding stability,” Case Studies in Construction Materials, Vol. 17, p. e01158, Dec. 2022, https://doi.org/10.1016/j.cscm.2022.e01158
-
J. Liu, C. Liao, H. Jin, Z. Jiang, D. Zhong, and L. Tang, “Numerical model of the effect of water vapor environment on the chloride transport in concrete,” Construction and Building Materials, Vol. 311, p. 125330, Dec. 2021, https://doi.org/10.1016/j.conbuildmat.2021.125330
-
J. Sun, Z. Jin, H. Chang, and W. Zhang, “A review of chloride transport in concrete exposed to the marine atmosphere zone environment: experiments and numerical models,” Journal of Building Engineering, Vol. 84, p. 108591, May 2024, https://doi.org/10.1016/j.jobe.2024.108591
-
G.-J. Yin, L.-B. Li, X.-D. Wen, L. Miao, S.-S. Wang, and X.-B. Zuo, “Numerical modelling on moisture and sulfate ion transport in unsaturated concrete slab under dry-wet cycles,” Journal of Building Engineering, Vol. 89, p. 109296, Jul. 2024, https://doi.org/10.1016/j.jobe.2024.109296
-
Y. Wang, S. Guo, B. Yan, Z. Liu, Y. Wang, and C. Yuan, “Experimental and analytical investigation on chloride ions transport in concrete considering the effect of dry-exposure ratio under diurnal tidal environment,” Construction and Building Materials, Vol. 328, p. 127138, Apr. 2022, https://doi.org/10.1016/j.conbuildmat.2022.127138
-
Z.-Q. Shan, G.-J. Yin, X.-D. Wen, L. Miao, S.-S. Wang, and X.-B. Zuo, “Numerical simulation on transport-crystallization-mechanical behavior in concrete structure under external sulfate attack and wetting-drying cycles,” Materials and Design, Vol. 241, p. 112908, May 2024, https://doi.org/10.1016/j.matdes.2024.112908
-
Q. Liu, L. Sun, X. Zhu, L. Xu, and G. Zhao, “Chloride transport in the reinforced concrete column under the marine environment: Distinguish the atmospheric, tidal-splash and submerged zones,” Structures, Vol. 39, pp. 365–377, May 2022, https://doi.org/10.1016/j.istruc.2022.03.041
-
Q. Zhang, J. He, L. Song, K. Hu, and P. Sun, “Theoretical model of water vapor absorption-desorption equilibrium of concrete considering the effect of temperature,” Construction and Building Materials, Vol. 375, p. 130968, Apr. 2023, https://doi.org/10.1016/j.conbuildmat.2023.130968
-
L. Dudi, S. Krishnan, and S. Bishnoi, “Numerical modeling for predicting service life of reinforced concrete structures exposed to chloride,” Journal of Building Engineering, Vol. 79, p. 107867, Nov. 2023, https://doi.org/10.1016/j.jobe.2023.107867
-
Q. Yuan, “Basic research on chloride ion transport test method in cement-based materials,” (in Chinese), Central South University, 2009.
-
A. Dousti, R. Rashetnia, B. Ahmadi, and M. Shekarchi, “Influence of exposure temperature on chloride diffusion in concretes incorporating silica fume or natural zeolite,” Construction and Building Materials, Vol. 49, pp. 393–399, Dec. 2013, https://doi.org/10.1016/j.conbuildmat.2013.08.086
About this article
The study was supported by Science and Technology Project of Guangdong Transportation Group (No. JT2020YB02), Key science and technology projects in transportation industry (No. 2020-MS1-004). The authors also express their acknowledgement to the research fund of Road& Bridge South China Engineering Co., Ltd. for their financial support.
The datasets generated during and/or analyzed during the current study are available from the corresponding author on reasonable request.
Guohe Guo: conceptualization, formal analysis, investigation, methodology, visualization, writing-original draft preparation. Lizhou Sun: supervision, writing-review and editing, resources. Shangchuan Zhao: conceptualization, writing-review and editing. Longlong Liu: writing -original draft preparation, methodology, investigation.
The authors declare that they have no conflict of interest.