Abstract
The subject of the studies presented in this paper is the fabrication of electrochemical geophone, especially the electrochemical transducer with symmetrical four-electrode cell by FPCB process technology. The geophone assembled by transducer, dumbbell-shaped tube, highly-flexible membranes, electrolyte solution and signal-amplification circuit, is calibrated using a standard vibration platform, and the results show a good consistency of each geophone parameters. Coupled with low cost, the electrochemical geophone by FPCB shows a good potential application prospect.
1. Introduction
Seismic exploration is one of the major means of obtaining underground information, and geophone is the indispensable component for sensing the ground vibration. With the exhausting of the surface layer resource, the resource exploration is developing towards increasing the exploration depth. For seismic exploration, the geophone should have a more sensitive ability, wider frequency bandwidth and bigger dynamic range to acquire the abundant information from the deeper underground, which makes it more like a low-version seismometer than in the past.
Now, the most general structure of a geophone is that a coil form is suspended around a permanent magnet by a pair of leaf springs, and the voltage is induced from the coil when it is moved in the Magnetic field. D. Yang et al. in literature [1] gave a detailed introduction about this moving-coil geophone, and pointed out the intrinsic shortcoming of frequency bandwidth. Normally, the high frequency limit is not more 30 times than the low frequency limit, which means that the high frequency limit will about 60 Hz if a geophone is selected with 2 Hz low frequency limit, and the bandwidth too narrow for the deep source detection. Also, the sensitivity of moving-coil geophone is not enough for sensing the weak vibration from the deeper underground. Although, the existing technologies, like force-balance capacitive transduction, are easy to solve upper problems, but the features of small size, low cost and good robustness are failed to meet the seismic exploration requirements due to the huge usage amount and heavy layout workload.
Electrochemical motion sensor substantially originated from 1990s, which should be attributed to the continuous research work from V. A. Kozlov and V. M. Agafonov et al. [2-7] The practical advantage of the electrochemical motion sensor is the ability for providing a high conversion factor with a very simple and suitable mass production signal converting system [7], which makes it possible for increasing the bandwidth by the mean of signal process or feedback control technology although the sensitivity for motion will be lost a little. So, there is a great potential for electrochemical motion sensor designed as geophone.
But when the electrochemical approach is used in geophone, there are two unavoidable problems for volume production, low cost and excellent consistency. The transducer with symmetrical four-electrode cell is the key unit. Now, there are two kinds of methods for the fabrication of conventional mechanics and modern MEMS. Conventional mechanics fabrication techniques suffer from the problem of misalignment between electrodes and insulation layers, which results in compromised performance, low production yield and therefore high cost[8].Modern MEMS techniques can enable proper alignment between electrodes and insulations[9-11], but strict environment foundation and complex processing is the huge obstacle for electrochemical sensor researchers without MEMS processing conditions.
In this paper, authors present an electrochemical transducer based on the FPCB (flexible printed circuit boards), which is widely used in the circuit boards process with extremely low cost and high efficiency. Then, the transducer is mounted in a designed tube to realize the structure of geophone, and the characteristic is evaluated by standard vibration platform.
2. Main text
2.1. Working principle
Similar with the previous studies [2-6], schematic depiction of electrochemical geophone is shown in Fig. 1. The dumbbell-shaped tube is generally designed for lowering the intrinsic frequency. Highly-flexible membranes are assembled at the both ends of the tube to seal the electrolyte, and also provide restoring force for the mechanical subsystem. Symmetrical four-electrode electrochemical cell is used as a sensing element, placed inside a channel filled with iodide salt aqueous solution with a small amount of tri-iodide. When the small electrical potential is applied between the electrodes, normally arrayed as ACCA (anode-cathode-cathode-anode), the highly sensitive current with the electrolyte solution motion is transduced.
Fig. 1Schematic depiction of electrochemical geophone
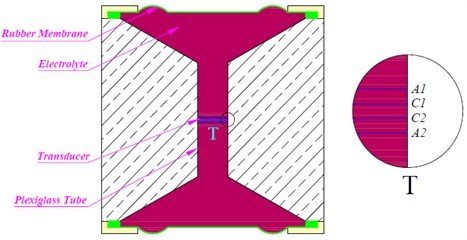
2.2. Fabrication and package
The electrochemical transducer was designed as shown in Fig. 2(a), and the electrodes were separated by dielectric spacers. Specifically, the electrodes were made by plain weave platinum metallic gauze (Purity 99.9 %, Nominal Aperture 0.12 mm, Wire diameter 0.04 mm, Wires/inch 152 and Open area 56 %). The dielectric spacers were processed by the three-layer reinforcement polyimide plates in FPCB, and each reinforcement polyimide plate has a strict thickness of 0.2 mm. Three dielectric spacers (Nominal Aperture 0.5 mm and Interval Thickness 0.2 mm) with proper alignment can be obtained by the one-time laser cutting of reinforcement polyimide plates. After being inserted among the dielectric spacers, the electrodes were bonded with the dielectric spacers by 0.0125 mm thermosetting film adhesive, and at last the electrodes were welded on the welding pad of FPCB. The dumbbell-shaped tube with the inside diameter of 5 mm was processed by acrylic, and the photograph of tube with the electrochemical transducer was shown as Fig. 2(b).
After a high precise 0.40 V electrical potential being loading on the anode electrodes, the signals from the two cathode electrodes were amplified by current amplifiers respectively. The sensor output could be obtained by differencing the two-cathode electrode amplified signals. In this experiment, amplifier MC33178 and comparator AD520 were selected for the requirement of high precise and low noise, and the circuit schematic was shown at Fig. 3.
Fig. 2a) schematic depiction of electrochemical transducer, b) photograph of tube with the electrochemical transducer
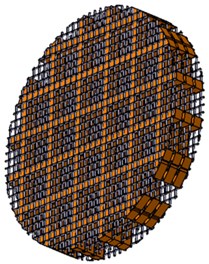
a)
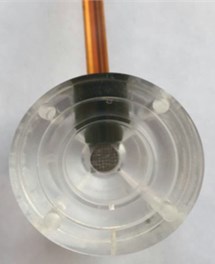
b)
Fig. 3The circuit schematic of electrochemical geophone
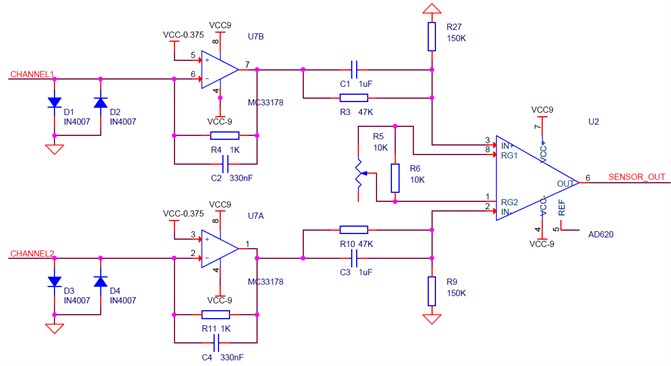
3. Results
The electrochemical geophone was accomplished by highly-flexible membranes sealing the ends of tube, injecting the electrolyte solution and connecting the signal-amplification circuit shown as Fig. 4. The overall dimensions with diameter of 35 mm and height of about 68 mm, is only a little longer than the conventional moving coil geophone SG-10 produced by SERCEL, which is widely used in seismic exploration.
Fig. 4The photograph of electrochemical geophone with highly-flexible membranes, electrolyte solution and signal-amplification circuit
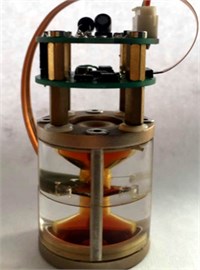
The primary parameters of electrochemical geophones were calibrated using a standard vibration platform, and three geophones were experimented to evaluate the consistency. The relative value of the geophone output should be noted for the reasons of the variability of the tri-iodide concentration, amplifiers gain, flexible membranes hardness and solution damping. The frequency response was calibrated at the vibration magnitude of 2 mm/s (Fig. 5(a)), and it was stopped at 150 Hz due to the high-frequency limit of the vibration platform. The uneven curves should be flattened by adjusting the circuit filter or adding a feedback structure in the next work. The velocity response was calibrated at the frequency of 16 Hz (Fig. 5(b)), and a linear relationship was obtained from 5 vibration magnitude points. The good consistency of three geophones was also observed from Fig. 5, which means that the process method of electrochemical transducer by FPCB is suitable for geophone.
Fig. 5a) Frequency response, b) velocity response curves of electrochemical geophones
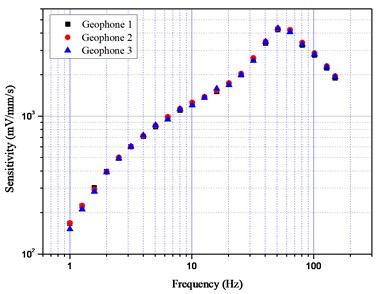
a)
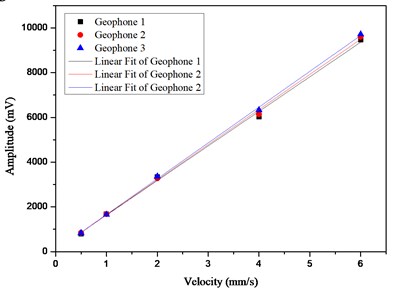
b)
4. Conclusions
An electrochemical geophone was fabricated based on FPCB processing technology, and frequency response and velocity response were calibrated using a standard vibration platform. The results showed the good consistency of each geophone parameters, which indicated the suitability of this method for geophone fabrication, although the frequency response curves were needed for further modification.
References
-
Yang D. P., Li N. R., Liu C. Y., Lin J. Improving the performance of a geophone through suspension system configuration. Review of Scientific Instruments, Vol. 85, Issue 12, 2014, p. 126104.
-
Kozlov V. A., Yaglom K. A. Fundamentals of liquid and solid-state measuring systems and data processing devices. MIPT Proceedings, Moscow, 1994, p. 37-42.
-
Kozlov V. A., Terent’ev D. A. Frequency characteristics of a spatially-confined electrochemical cell under conditions of convective diffusion. Russian Journal of Electrochemistry, Vol. 38, Issue 9, 2002, p. 992-999.
-
Zakharov I. S., Kozlov V. A. Stationary convective diffusion and nonlinear effects in an electrochemical transducer. Russian Journal of Electrochemistry, Vol. 39, Issue 4, 2003, p. 397-400.
-
Kozlov V. A., Agafonov V. M. Convective Accelerometer. U.S. Patent, 20050257616A1, 2005.
-
Agafonov V. M., Nesterov A. S. Convective current in a four-electrode electrochemical cell at various boundary conditions at anode. Russian Journal of Electrochemistry, Vol. 41, Issue 8, 2005, p. 880-884.
-
Krishtop V. G., Agafonov V. M., Bugaev A. S. Technological principles of motion parameter transducers based on mass and charge transport in electrochemical microsystems. Russian Journal of Electrochemistry, Vol. 48, Issue 7, 2012, p. 746-755.
-
Li G. B., Chen D. Y., Wang J. B., Jian C., He W. T., Fan Y. J., Deng T. A MEMS based seismic sensor using the electrochemical approach. Procedia Engineering, Vol. 47, 2012, p. 362-365.
-
Li G. B., Chen D. Y., He W. T., Wang J. B. Micro-machined electrochemical seismic sensors with interdigital electrodes. Key Engineering Materials, Vol. 503, 2012, p. 61-66.
-
He W. T., Chen D. Y., Li G. B., Wang J. B. Low frequency electrochemical accelerometer with low noise based on MEMS. Key Engineering Materials, Vol. 503, 2012, p. 75-80.
-
He W. T., Chen D. Y., Wang J. B., Zhang Z. Y. MEMS based broadband electrochemical seismometer. Optics and Precision Engineering, Vol. 23, Issue 2, 2015, p. 444-451.
Cited by
About this article
This research was supported by the National Science Foundation of Jilin Province, China (Grant No. 20140101001JC and 20170101088JC), and the National Science Foundation of China (Grant No. 41404139).