Abstract
The aim of this study to investigate the dynamic properties of luffa cylindrica fiber reinforced bio-composite as alternative natural composite materials. As dynamic properties resonance frequencies and damping measured both for the bio-composite and chemical composite materials. To able to compare the properties of natural fiber and chemical fiber, used the same matrix to produce the composite materials. Luffa cylindrica fiber and glass fiber were used as reinforcements, and epoxy resin was used as a matrix to make up the composite specimens. As a dynamic test, vibration tests were fulfilled using hummer. All results obviously showed that damping characteristics of Luffa cylindrica composite is higher than glass fiber composite materials. These results demonstrated that, natural fiber materials can be used instead of chemical materials, in absorbing the vibration due to their high damping.
1. Introduction
Recently, human beings have realized that unless the nature is protected, natural sources will be depleted and green areas, which are the sources of fresh air, will shrink. Composite materials that are produced by using petroleum base fiber materials (such as carbon fiber and glass fiber) are commonly used especially in automotive, space and aviation industries because of their high strength, corrosion resistance and low density properties. To lessen the usage of chemical fibers, the research on the ecological fibers are urgent so that to save the planet. To increase the applications of the environmental friendly materials, not only new materials should find but also their all characteristic properties must identify and upgrade.
The fact that plastic composite materials cannot decompose in the soil after being dumped is really hazardous to the nature. In this view, it will be a great step if the use of renewable bio-composite materials in industrial products does become more common. That’s why bio-composite materials have recently become the focus of the science of composite materials. Bodros et al. [1] presents investigation about natural fiber-biopolymer composites in order to determine whether or not bio-composites may replace glass fiber reinforced unsaturated polyester resins.
The major bio-materials that flax, jute hemp, kenaf and sisal are investigated in many studies. Despite the challenges, such as cultivation and continuity of these plants based materials, enhanced features are gaining immense importance. Also, Bos et al. [2] underlined this point The use of natural fibers for the reinforcement of composites has lately received increasing attention, both by the academia and by the industry” in their study.
In previous studies, Kocak [3] studied Luffa cylindrica fiber with different chemical processes using conventional and ultrasonic processes to identify the behaviors of this materials. As proposed in this study that the best chemical treatment are as formic acid and acetic acid using ultrasonic processes. Shen et al. [4] presents in their study, a series of compressive tests to examine the stiffness, strength and energy absorption characteristics of the luffa sponge material under quasi-static compressive load. Demir et al. [5] investigate in their studied that using natural Luffa cylindrica fibers as adsorbent removal of methylene blue dye from aqueous solutions at different temperatures and dye concentrations. They focused on the thermodynamics and kinetics of adsorption characteristics of this material. Boynard and D’Almeida [6] used the sponge gourd (Luffa cylindrica) as reinforcement in resin matrix composite materials. They investigate the morphology of the fibrous vascular system of Luffa’s fruit and mechanical properties. In this study underlined that without any surface treatment, Luffa already has a high potential use as a core material in hybrid composites. Ghali et al. [7] present in their study that the Luffa fibres were treated by tow methods: alkali treatment and mixed treatment (sodium hydroxide and hydrogen peroxide). According the present result the alkali treatment (120 °C; 3 h; 4 % NaOH) shows a good cleaning and the higher crystallinity index of treated fibres. Paglicawan et al. [8] studied the flexural properties of loofah fiber reinforced plastic using polyester resin as a matrix. According the result of this study the concentration of fibers in the loofah itself has a significant effect on the mechanical properties of transverse and longitudinal direction. The tensile and flexural modulus varied depending on the sample preparation of the composite and the direction of loading. Kocak et al. [9] examine that, mechanical properties of Luffa cylindrica fibers how effect from the chemical modification. And they offered to use formic acid and acetic acid on the modification of these materials. Satyanarayana et al. [10] presents an overview in their study of the developments made in the area of biodegradable composites, in terms of market, processing methods, matrix-reinforcement systems, morphology, properties and product development. Missoum et al. [11] studied the vibration tests to identify the elasticity modulus in two directions of composite materials glass/polyester. Qian et al. [12] studied a method for identifying elastic and damping properties of composite laminates by using vibration test data. The development of ecofriendly instead of chemical materials is vital due to environmental reasons. Thus, reducing the use of petroleum-based materials that are harmful to planet and increasing the use of bio-materials instead will be realized in the future.
In this study the Luffa cylindrica fiber (because of its resistance in dry state and the mated structure of its fibers) that is grown in the southern of Turkey is thought to be an alternative as a natural based bio-composite to petroleum based glass fiber composite. Because there isn’t any study on the dynamic properties of Luffa cylindrica fiber, the necessary dynamic properties of this item will be determined and will be added to the literature. In order to be able to determine these properties of these bio-composite materials vibration tests will be achieved. Under the same production parameters glass fiber composite will be produced and the same tests will be carried out. According to the results the strength of Luffa cylindrica fiber will be compared to the strength of glass fiber. In the event of the determined features of the Luffa cylindrica fiber bio-composite being superior to other alternatives in proportion; especially the potential area of usage, when thought in aero-plane, automotive, yacht and decoration sectors, is provided for making a great contribution to save the planet and to enhance the environmental friendly materials. Because of that, Luffa cylindrica fiber studied to determinate their dynamic properties likes damping and resonance frequencies by modal testing.
Fig. 1Stage of Luffa cylindrica
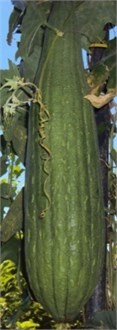
a)
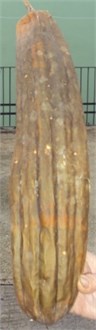
b)
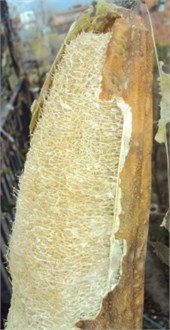
c)
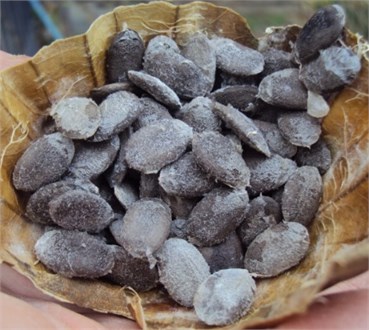
d)
2. Materials and specimens
The reinforcement materials used in this study are a commercial Luffa cylindrica fiber of Mediterranean variety and woven type glass fiber. The glass fiber roller is made of a double woven-ply with fiber arrangement of 0° to 90° with respect to the roller axis. It is obligation to use the same matrix while comparing the properties of natural fiber with chemical fiber. Therefore, epoxy resin (DURATEK 1200) was used as a matrix to make up the composite specimens.
Luffa Cylindrica, which is shown in Fig. 1, firstly has a form of a fruit which is covered with green peel (Fig. 1(a)) and hanging on the plant. When the ripening period of fibers that inside the fruit is complete, the outer green layer (Fig. 1(b)) starts to dry. When the dry layer is removed as it is shown in Fig. 1(c), the fibrous structure is emerging. There are about 4 or 5 pieces of fruit in each Luffa Cylindrica plant and about 50 pieces of the core in each fruit, as can be seen from Fig. 1(d), that can be used as seed.
3. Experiments
3.1. Preparation of the test specimens
In this study, the dynamic properties of the bio-composite material that is strengthened with the addition of Luffa cylindrica fiber will be determined and compared with other properties of alternative natural fibers and fiber glasses. Luffa fibers are prepared without any surface treatment to observe the potential use as a core material in hybrid composites as described in the literature [6].
Fig. 2Luffa cylindrica structure in a dry state
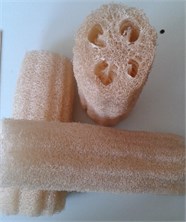
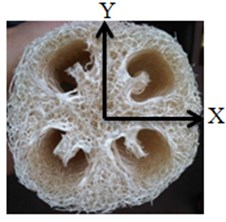
As shown in Fig. 2 specimens compressed on the Y axis using hydraulic press machine which have heated plates to able to make useful stage for curing the epoxy resin. The lower plate is fixed to the machine frame while the upper plate is controlled by a PLC (Programmable Logic Controller) system of 5 bars pressure, to compress the stacked laminates. And the heat is adjusted to 80 °C during the curing process about 300 minutes. Produced composite beams are shown in Fig. 3.
Fig. 3Produced bio-composite beams
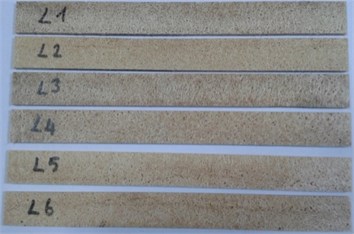
It should be underlined that the curing time, the curing temperature and the pressure are more important parameters to obtain the similar composite specimens. Therefore, the entire specimen are produced under the same conditions.
The properties of the composite materials are shown in Table 1. For the analytical calculations the theory of the rules of mixture is taken as reference [13].
Even though, the Young’s moduli of Luffa cylindrica fiber composite lower than glass fiber composite the low density of this material are considerable significant for which in the applications needs low density (Table 1).
Table 1Properties of composite materials
Glass Fiber (GF) composite | Luffa Cylindrica Fiber (LCF) composite | |||||
GF | Epoxy resin | Analytical calculation | LCF | Epoxy resin | Analytical calculation | |
Density (kg/m3) | 1797.8 | 1150 | 1331.4 | 983 | 1150 | 1086.5 |
Young modulus (GPa) | 74 | 3.5 | 23.2 | 30 | 3.5 | 13.6 |
Volume fraction (%) | 0.28 | 0.72 | 1.0 | 0.38 | 0.62 | 1.0 |
3.2. Vibration tests
Impact tests are conducted to determine the natural frequencies and modal damping levels (loss factors) of the composite structures. For this purpose, a few frequency response functions for various impact and response points of the test samples in the free-free boundary conditions are measured. As shown in Fig. 4, the test samples are excited using a modal hammer (Endevco 2302-50) and the response to the excitation is measured using an accelerometer (Bruel&Kjaer 4507B). The frequency response functions with minimal mass loading effects are used to identify the natural frequencies and loss factors [14, 15].
Fig. 4Schematic test setup
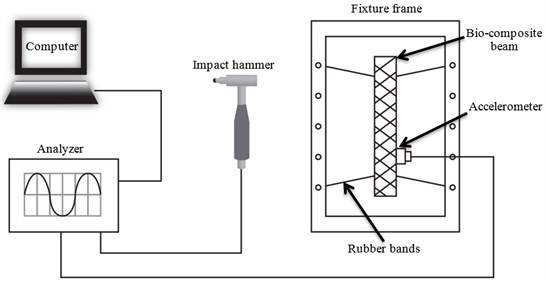
Table 2The natural frequencies and loss factors of glass (GFC) and Luffa cylindrica fiber composite (LCFC) materials
Mode no. | GFC | LCFC | ||
Frequency (Hz) | Loss factor (η) | Frequency (Hz) | Loss factor (η) | |
1 | 301.5 | 0.004 | 159.0 | 0.008 |
2 | 813.6 | 0.005 | 419.0 | 0.012 |
3 | 1579.3 | 0.006 | 862.0 | 0.015 |
Average | 0.005 | 0.012 |
As a modal identification methods, the line-fit method is proved to be quite successful for identification damping levels on measured frequency response functions [16] and it is widely used in the literature for this purpose [17, 18]. Therefore, modal loss factors are identified using line fit method in this study [15]. Overall, the natural frequencies and loss factors of the glass and luffa specimens are listed in Table 2.
3.2.1. Determination the Young’s modulus
Composite structures are widely used in the industry because of their high strength and low weight properties. Particularly, in automotive industry some applications of composite materials are used around the engine, such as internal engine covers [17]. Vibration tests were carried out for composite beams under Free - Free fixation type condition to determine the elasticity modulus.
Fig. 5Dimensions of the test specimens
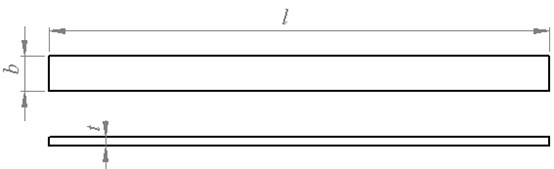
The test specimens are cut according the dimensions that shown in Fig. 5. The Young’s modulus was calculated by the Eq. (1) [18] using measured resonance frequency of composite materials:
where fn is measured frequency of nth mode by examining the maxima of the amplitude or phase change of the measured frequency response functions, X2n; constant for nth mode that depends on boundary condition [18], l; length of specimen from the fixation point, E; Young’s modulus, I; inertia moment (bt3/12), ρ; density and s; cross sectional area of beam (b.t).
The thicknesses (t) for Luffa cylindrica and glass composite specimens are 3.9 mm and 4.0 mm. The wideness (b) and the length (l) of specimens are 15.1 mm and 212 mm respectively. Young’s modulus is calculated by Eq. (1) using measured resonance frequency. Vibration test results of glass and Luffa cylindrica composite materials are given in Table 3.
Table 3Vibration test results of Young’s modulus of glass (GFC) and Luffa cylindrica fiber composite (LCFC) materials (standard deviations in brackets)
Mode no. | Frequency (Hz) | Young’s modulus (GPa) | Average Young’s modulus (GPa) | |||
GFC | LCFC | GFC | LCFC | GFC | LCFC | |
1 | 192.0 | 159.0 | 24.9 | 9.2 | 15.8 (8.4) | 9.0 (0.5) |
2 | 303.0 | 419.0 | 8.2 | 8.4 | ||
3 | 792.0 | 862.0 | 14.5 | 9.3 |
According the impact modal testing, the elastic modulus of glass fiber composite (15.8 GPa) is higher than Luffa cylindrica fiber composite (9 GPa) materials.
3.2.2. Determination of the damping
Fiber reinforced composite parts that are used around the engine must have high damping characteristics to absorb the vibration that is revealed from the engine. The damping properties are calculated according to the line fit-method [18] of measured resonance frequencies:
where f1 is measured resonance frequency (f1< 200 Hz), f2; measured resonance frequency (f2> 200 Hz), d1; measured modal damping on f1 and d2; measured modal damping on f2.
The damping (d200) values are calculated by the Eq. (2) to compare the damping properties of glass and Luffa cylindrica composite materials. According to the standard [19], resonance frequencies that are greater and smaller than 200 Hz (f2> 200 >f1) should be used to calculate d200 values.
As given in Table 4, the results demonstrate that the damping of Luffa cylindrica fiber composite is very high than glass fiber composite materials. Natural fiber composite materials have significant damping characteristics than the chemical fiber composites.
Table 4Vibration test results of damping of Glass (GFC) and Luffa cylindrica fiber composite (LCFC) materials
Mode no. | Frequency (Hz) | Damping (%) | Damping (d200) | |||
GFC | LCFC | GFC | LCFC | GFC | LCFC | |
1 | 192.0 | 159.0 | 0.4 | 0.8 | 0.41 | 0.86 |
2 | 303.0 | 419.0 | 0.5 | 1.2 | ||
3 | 792.0 | 862.0 | 0.6 | 1.5 |
4. Results and analysis
4.1. Young’s modulus
In this section of the study, experimental Young’s modulus of Luffa cylindrica and glass composite materials, which is obtained by vibration, is compared.
Fig. 6Comparison of the calculated Young’s modulus of Glass (GFC) and Luffa cylindrica fiber composite (LCFC) materials
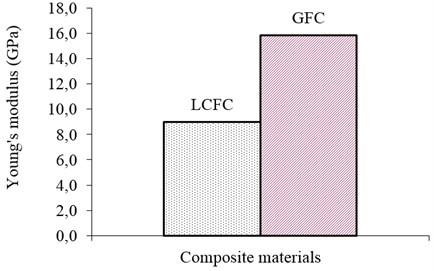
Fig. 7Comparison of the experimental damping of Glass (GFC) and Luffa cylindrica fiber composite (LCFC) materials
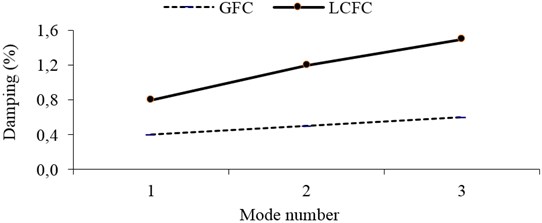
As given in Fig. 6, Young’s modulus of glass fiber and Luffa cylindrica fiber composite material are calculated as 11.4 GPa and 9.8 GPa from vibration test respectively. These obtained results clearly reflect that the strength of glass fiber composite is higher than the Luffa cylindrica fiber composite under vibration excitation.
4.2. Damping characteristics
In this section of the study, the damping characteristic of Luffa cylindrica and glass composite, which determines the absorption properties of the materials, is compared.
As shown in Fig. 7, the damping characteristic of plant based materials is clearly higher than the petroleum based materials under impact loading. When considered the working condition of the engine, the damping properties of structure that will be used around the engine become crucial.
5. Conclusion
The objective of this study is to find alternative green composite materials that will be used instead of petroleum-based materials. As underlined Shen and et al. [20] Luffa sponge has the potential to be used as an alternative sustainable material for various engineering applications such as packaging, acoustic and vibration isolation, and impact energy absorption.
According to obtained results, damping characteristic of Luffa cylindrica fiber composite materials is higher than glass fiber composite materials. Also, the damping properties of Luffa cylindrica fiber more effective than flax fiber [17] as natural reinforcement. Therefore, this result is significant to find alternative green material. These results demonstrate that plant based fibers can replace petroleum based fibers as a reinforcement in applications that the structures are exposed to vibration excitation, and in applications where the absorption of vibration has major importance.
References
-
Bodros E., Pillin I., Montrelay N., Baley C. Could biopolymers reinforced by randomly scattered flax fibre be used in structural applications? Composites Science and Technology, Vol. 67, Issue 3-4, 2007, p. 462-470.
-
Bos H. L., Müssig J., Oever V. D., Martien J. A. Mechanical properties of short-flax-fibre reinforced compounds. Composites Part A: Applied Science and Manufacturing, Vol. 37, Issue 10, 2006, p. 1591-1604.
-
Kocak D. The study of the effects of different chemical compounds applied on luffa cylindrica fibres with the help of ultrasonic energy. Journal of Polymer Engineering, Vol. 28, Issue 8, 2008, p. 501-515.
-
Shen J., Min Xie Y., Huang X., Zhou S., Ruan D. Mechanical properties of Luffa sponge. Journal of the Mechanical Behavior of Biomedical Materials, Vol. 15, 2012, p. 141-152.
-
Demir H., Top A., Balköse D., Ülkü S. Dye adsorption behavior of Luffa cylindrica fibers. Journal of Hazardous Materials, Vol. 153, Issue 1-2, 2008, p. 389-394.
-
Boynard C. A., D’Almeida J. R. M. Morphological characterization and mechanical behavior of sponge gourd (Luffa cylindrica) – polyester composite materials. Polymer-Plastics Technology and Engineering, Vol. 39, Issue 3, 2000, p. 489-499.
-
Ghali L., Msahli S., Zidi M., Sakli F. Effect of pre-treatment of Luffa fibres on the structural properties. Materials Letters, Vol. 63, Issue 1, 2009, p. 61-63.
-
Paglicawan M. A., Cabillon M. S., Cerbito R. P., Santos E. O. Loofah fiber as reinforcement material for composite. Philippine Journal of Science, Vol.134, Issue 2, 2005, p. 113-120.
-
Kocak D., Merdan N., Yuksek M., Sancak E. Effects of chemical modifications on mechanical properties of Luffa cylindrica. Asian Journal of Chemistry, Vol. 25, Issue 2, 2013, p. 637-641.
-
Satyanarayana K. G., Arizaga G. G. C., Wypych F. Biodegradable composites based on lignocellulosic fibers – an overview. Progress in Polymer Science, Vol. 34, Issue 9, 2009, p. 982-1021.
-
Missoum L., Labbaci B., Djermane M., Moudden B., Abdeldjebar R. Identification of Young modulus by a vibration experimental method. Premier Collogue International IMPACT 2010, Djerba, Tunisie, 2010.
-
Qian G.-L., Hoa S. V., Xiao X. A Vibration method for measuring mechanical properties of composite, theory and experiment. Composite Structures, Vol. 39, Issues 1-2, 1997, p. 31-38.
-
Jones R. Mechanics of Composite Materials. Second Edition. Taylor and Franci Inc., Blacksburg, Virginia, USA, 1999, p. 126-135.
-
Koruk H. Quantification and minimization of sensor effects on modal parameters of lightweight structures. Journal of Vibroengineering, Vol. 16, Issue 4, 2014, p. 1952-1963.
-
Koruk H., Dreyer J. T., Singh R. Modal analysis of thin cylindrical shells with cardboard liners and estimation of loss factors. Mechanical Systems and Signal Processing, Vol. 45, Issue 2, 2014, p. 346-359.
-
Sanliturk K. Y., Koruk H. Development and validation of a composite finite element with damping capability. Composite Structures, Vol. 97, 2013, p. 136-146.
-
Genc G., El Hafidi A., Gning P. B. Comparison of the mechanical properties of flax and glass fiber composite materials. Journal of Vibroengineering, Vol. 14, Issue 2, 2012, p. 572-581.
-
Koruk H., Sanliturk K. Y. Damping uncertainty due to noise and exponential windowing. Journal of Sound and Vibration, Vol. 330, Issue 23, 2011, p. 5690-5706.
-
D 45 1809 Standard. Amortissants Vibratoires Mesure Du Facture D’amortissement (Méthode Oberst). Renault Automobiles, 1992.
-
Shen J., Xie Y. M., Huang X., Zhou S., Ruan D. Behaviour of Luffa sponge material under dynamic loading. International Journal of Impact Engineering, Vol. 57, 2013, p. 17-26.
About this article
This research is supported by Marmara University, Scientific Research Projects Committee (BAPKO) with Grant Number FEN-A-110913-0377. I would like to express my great appreciation to Dr. Hasan Koruk (Istanbul Technical University) and Prof. Dr. Nihat Akkus (Marmara University) for their valuable and constructive suggestions during the planning and development of this work.