Abstract
The aim of current study is to determine the hydrodynamic characteristics of swimmer’s hand models with different shapes, for various combinations of its orientation and velocity, simulating propulsive phase of front crawl swimming. A real swimmer’s hand with closed and separated fingers was scanned using 3D scanner. The fluid flow simulation was carried out using ANSYS FLUENT®, for the investigation of hydrodynamic parameter's distribution around the swimmer's hand model. Drag and lift forces were calculated using computational fluid dynamics in steady state. The study demonstrates that, in all cases hand model with separated fingers generates higher drag force than hand model with closed finger's. Therefore hand model with separated fingers is able to produce higher forward propulsion in propulsive phase of front crawl swimming.
1. Introduction
Propulsion is one of the key factors determining performance in human competitive front crawl swimming [1] and the main part – about 85 % to 90 % of propulsion force is produced by the swimmer's arm movements [2, 3]. Several studies maintain that relatively higher propulsion force is generated by hands [1, 4] when compared with upper arm.
Propulsive force is taken as vector sum of lift (FL) and drag (FD) forces, which are dependent on density of the fluid, square of velocity and surface area of hand (i.e. projected frontal area of hand), coefficient of lift (CL) and drag (CD), which vary according to the shape of the limb and its orientation with respect to main flow direction. The angle of attack or pitch angle (defined as inclination of the propelling surface to its direction of motion) and the sweepback angle (showing leading edge of the hand) define the orientation of the hand.
The orientation of hand is one of the factors which contributes to propulsive force. The ideal pitch angle during the underwater motion of the hand will produce an optimal combination of lift and drag forces, which in turn will generate a resultant force, which is predominantly directed – forward [5]. At high Reynolds numbers (which the swimmers usually undergo during the competitions) two main factors affect drag force: shape and arm orientation [6].
The different methods were used to analyze different shapes of the hand and its contribution to the lift and drag forces. Steady-state computational fluid dynamics (CFD) method was applied to analyze the hydrodynamic characteristics of a real geometric models of swimmer's hand with the thumb oriented in different positions keeping the fingers closed [7]. The analysis of present study reveals that the hand model with the thumb fully abducted offered higher drag values than the hand model with the thumb partially abducted and adducted at angles of attack of 0° and 45° (the sweepback angle equal 0). An optimal finger spacing (12°, roughly corresponding to the resting hand posture) gives rise to the drag coefficient (+8.8 %), which is ‘functionally equivalent’ to a greater hand palm area, thus leading to a lower stroke frequency producing same amount of thrust, which benefits muscles, also generously augmenting hydraulic and propulsive efficiencies [8].
However, to our knowledge, present study is novel and till date no previous published research is found, which apply CFD method for the complete analysis of hydrodynamic parameters acting on different hand models and simulating the realistic propulsive front crawl stroke by the variation of both angles of hand orientation.
2. Methods
The left hand of an international level female swimmer was scanned with 3D scanner Artec L. Two nodal 3D models of hand with closed and with separated fingers were created and transferred to the surfacing software Leios2 to optimize and transform the point cloud into a complete 3D polygon models (Fig. 1).
Fig. 1Hand model with a) closed fingers, b) separated fingers
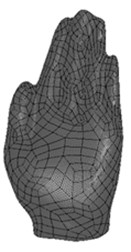
a)
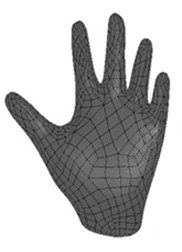
b)
Initial kinematic parameters (Table 1) corresponding to real swimmer’s front crawl propulsion phase were obtained from previous experimental study [9]. Both models of the hand were oriented into six different positions with the combinations of various pitch and sweepback angles. Different values of average hand velocity were taken from experiment [9] and in CFD calculation this velocity was used as the initial velocity of water flow while the hand model was kept stationary.
Table 1Initial experimental kinematic data [9]
Position | Pitch angles (deg.) | Sweepback angle (deg.) | Velocity (m/s) |
1 | 44.37 | 127.23 | 1.79 |
2 | 55.46 | 192.20 | 1.82 |
3 | 42.89 | 295.06 | 2.01 |
4 | 36.98 | 243.63 | 2.20 |
5 | 25.14 | 232.80 | 2.53 |
6 | 20.71 | 232.80 | 2.75 |
CFD method, based on the Navier-Stokes equations, was used to simulate drag and lift forces of the hand. Steady-state flow simulation was implemented by keeping hand static in a meshed domain with the fluid flowing at constant velocity. The boundary conditions were set: initial velocity was determined on the left side of the vertical surface of domain; pressure was set equal to 0 Pa on the right side of the vertical surface of domain; symmetry was considered on the remaining side faces and bottom of the domain.
All the numerical computational schemes considered were of second-order, which provides a more accurate solution than first-order schemes. Incompressible flow was assumed with turbulence intensity of 1.0 % and turbulence scale of 0.10 m. The water temperature was 28°C with a density of 998.2 kg·m-3, viscosity of 0.001 kg-1·m·s-1 and acceleration due to gravity of 9.81 m·s-2.
3. Results
Drag and lift forces were analyzed for different water flow velocity and orientation of the hand for both hand models: fingers closed and fingers separated. Drag force is higher (except second position of hand orientation) and lift force is lower for the hand model with separated fingers when compared with hand model with closed fingers during entire propulsive phase (Fig. 2). The means of drag and lift forces are quiet similar in every propulsion phase, while using hand model with closed fingers, though, drag force is apparently higher than lift force for the hand model with separated fingers (Fig. 3).
Fig. 2a) Drag and b) lift forces versus different hand position (orientation) acting on hand model with closed and separated fingers with respective increment of velocity
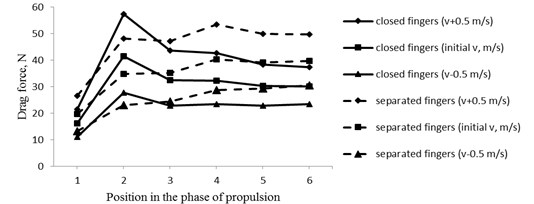
a)
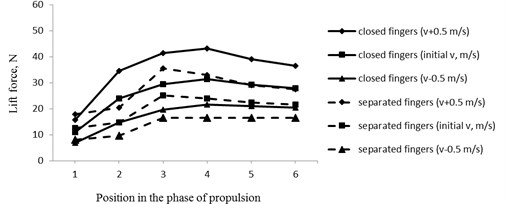
b)
Fig. 3Drag (FD) and lift (FL) forces versus different hand position (orientation) acting on hand model with a) closed fingers and b) separated fingers with respective increment of velocity
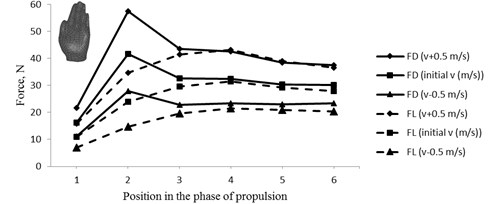
a)
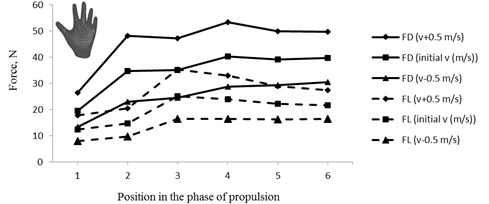
b)
4. Conclusions
Hand model with separated fingers are more valuable than hand model with closed fingers for all orientations of the hand and for entire range of water flow velocities due to production of higher drag force, which is crucial for a swimmer to generate higher forward propulsion.
References
-
Toussaint H., Truijens M. Biomechanical aspects of peak performance in human swimming. Animal Biology, Vol. 55, Issue 1, 2005, p. 17-40.
-
Hollander A. P., de Groot G., van Ingen Schenau G., Kahman R., Toussaint H. Contribution of the legs to propulsion in front crawl swimming. Swimming Science V, Illinois, Human Kinetics Books, 1988.
-
Deschodt V. Relative contribution of arms and legs in human to propulsion in 25 m sprint front crawl swimming. European Journal of Applied Physiology, Vol. 80, Issue 3, 1999, p. 192-199.
-
Butovich N. A., Chudovsky V. D. Sport Swimming. Biomechanics in Sport. Oxford, Blackwell Science, 1968.
-
Schleihauf R. E. Biomechanics Of Human Movement. Authorhouse Author Solutions, Inc. Indiana, 2004.
-
Gardano P., Dabnichki P. On hydrodynamics of drag and lift of the human arm. Journal of Biomechanics, Vol. 39, Issue 15, 2006, p. 2767-2773.
-
Marinho D. A., Rouboa A. I., Alves F. B., Vilas-Boas J. P., Machado L., Reis V. M., Silva A. J. Hydrodynamic analysis of different thumb positions in swimming. Journal of Sports Science and Medicine, Vol. 8, Issue 1, 2009, p. 58-66.
-
Minetti A. E., Machtsiras G., Masters J. C. The optimum finger spacing in human swimming. Journal of Biomechanics, Vol. 42, Issue 13, 2009, p. 2188-2190.
-
Gourgoulis V., Antoniou P., Aggeloussis N., Mavridis G., Kasimatis P., Vezos N., Boli A., Mavromatis G. Kinematic characteristics of the stroke and orientation of the hand during front crawl resisted swimming. Journal of Sports Sciences, Vol. 28, Issue 11, 2010, p. 1165-1173.