Abstract
Electromagnetic effect during crystallization of melts of various materials (metallic, salt, slag, oxide) is able to change significantly its macro- and microstructure and improve its physical and technical characteristics. There are many hypotheses about the mechanism of electromagnetic field effect on the structure of various metals. There are limited studies on the effect of electric, magnetic and temperature fields on the crystallization of non-metallic crystals in complex halide and oxide systems. The available works on this topic are fragmentary and are aimed only at solving the utilitarian problem of obtaining crystals. No studies on the mechanism of electromagnetic field effect during high-temperature crystallization of oxide refractory melts on its final characteristics have been found in the known scientific literature. In this paper, for the first time, based on the analysis of known hypotheses, the new study of mineral composition, structure-sensitive characteristics and structural units of melts, new hypothesis about the mechanism of effect of alternating electromagnetic field on the structure of oxide refractory materials crystallizing from melts on the example of forsterite, forsterite-chromite and corundum refractories has been put forward. The most probable effect mechanism of an alternating electromagnetic field on the structure of crystallizing oxide refractory castings is its periodically orienting and intensifying migration of the structural units of the melt action.
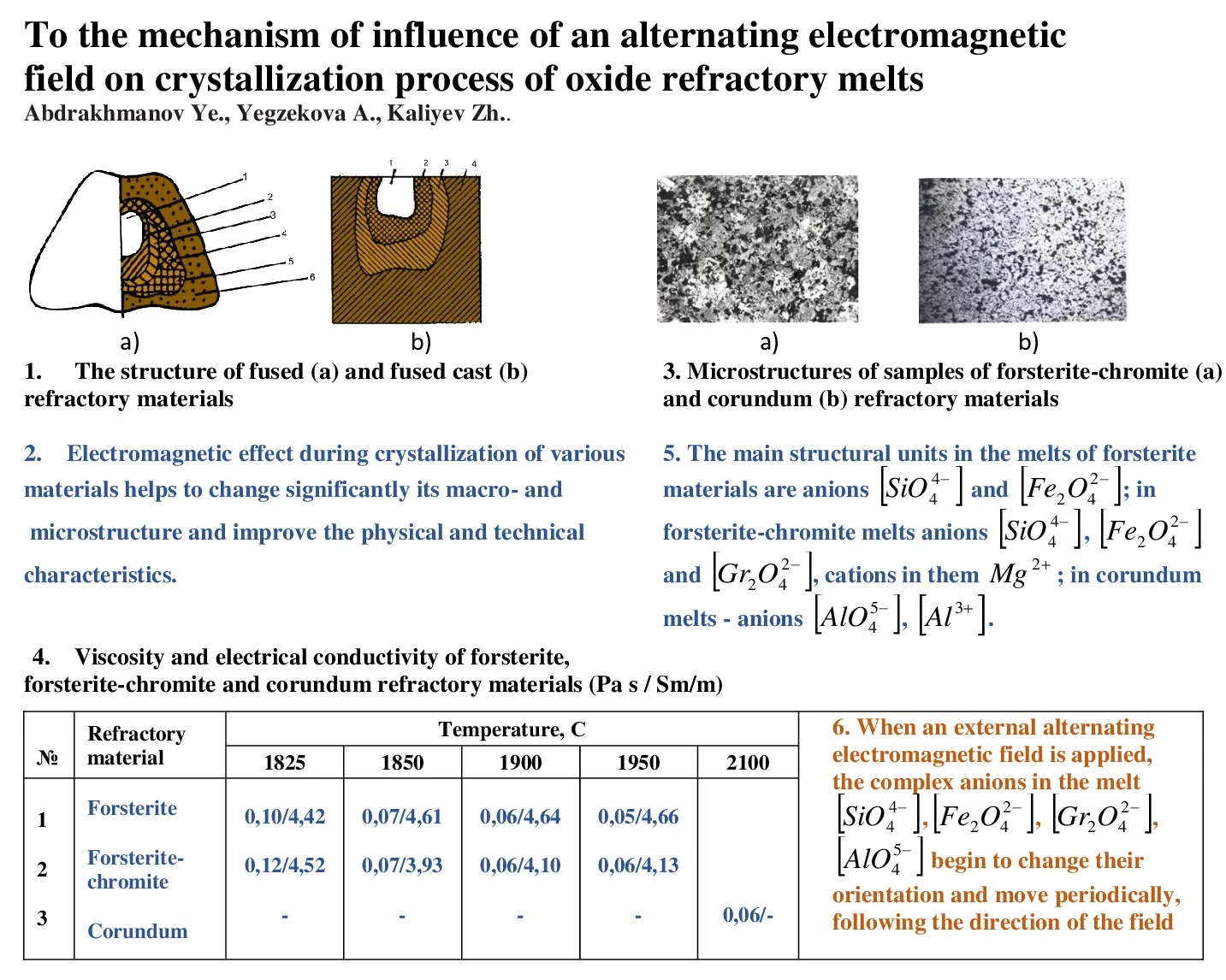
Highlights
- Petrographic analysis of mineral composition has been carried out.
- first time time viscosity and electrical conductivity of the melts have been studied and the structural units of forsterite, forsterite-chromite and Corundum refractory melts at high temperatures up to 1950 C have been established.
- First time time the mechanism of alternating electromagnetic field effect on crystallization process based on periodical orienting influence of electromagnetic field on melt particles and acceleration of particles transport to crystallization front has been proposed.
1. Introduction
Electromagnetic effect during crystallization of various materials, as shown by a number of experimental studies [1-3], including those conducted by the authors of this work [4-7], helps to change significantly its macro- and microstructure and improve the physical and technical characteristics.
Electromagnetic exposure eliminates the pronounced zoning of the structure characteristic of melted and melt-molded refractories. The castings produced under normal conditions have distinctly different zones: the “crust”, the thin, fine-grained part; the porous zone, with a coarse-grained columnar crystallization of phases, with pores extending towards the center of the casting; the transition dense zone (Fig. 1).
Electromagnetically treated castings are characterized by a homogeneous dense structure with a small number of pores distributed throughout the casting. The density of forsterite castings increases from 2.215 103 kg/m3 to 2.514 103 kg/m3, i.e. by 13 %. The structure of forsterite-chromite blocks obtained with electromagnetic effect during crystallization changes essentially. In the block volume the size of crystals of chromium spinelide decreases and does not exceed twofold size of the same small crystals near the lateral walls. Forsterite crystals have pronounced cleavage. Channel pores and open porosity of (15-18) %, typical for conventional blocks, under the effect of electromagnetic fields turn into dead-end pores with a decrease in open porosity to (11-12) %. In corundum castings exposed to electromagnetic fields during drainage and crystallization of the melt, unlike conventional ones, a macrostructure is formed with fine pores evenly distributed over the casting volume and without visible cracks.
Fig. 1a) The structure of fused: 1 – central zone; 2 – peripheral zone; 3 – zone of single crystals; 4 – side crust; 5 – lower crust; 6 – scree (undermelted) and b) fused cast refractory materials: 1 – shrink shell; 2 – moss zone; 3 – porous zone; 4 – dense zone
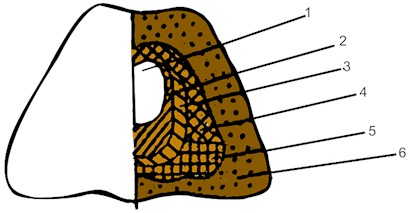
a)
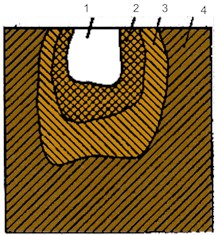
b)
However, a clear explanation of the mechanism of electromagnetic field effect on the formation process of oxide refractory castings is not available at the moment. The lack of theoretical foundations of electromagnetic treatment of oxide refractory materials hinders the development of this direction, reducing it to a technical technique, the feasibility of which is tested by trial and error. Therefore, the study of the mechanism of electromagnetic field effect on the structures of crystallizing melts of oxide refractory materials is one of the urgent tasks.
Research analysis in the metallurgy field shows that most authors, when explaining the characteristics of liquid metals and alloys, rely on the notion of its cluster structure [8]. A cluster is a crystal-like thickening of atoms, around which there exists a zone of disordered atoms of no more than 3...5 %, which ensures melt fluidity. The authors in the work [8] succeeded in explaining the forming structure of ingots, based on the cluster mechanism of the crystallization process of liquid metals and alloys. According to their hypothesis, superheated liquid metal in the head part of the bath, which has smaller clusters, moves under the effect of magnetic fields to its tail part, and provides refinement of the primary structure of the weld metal.
The use of controlling effects of longitudinal magnetic fields, or transverse magnetic fields in arc welding increases the productivity of the process, refines the structure of welds, and improves the service characteristics of welded products [9-12]. In [12] it is shown that there are many hypotheses about the mechanism of weld structure refinement in welding with controlling magnetic fields. Grinding of structural components of weld metal in welding with magnetic effect probably occurs at the stage of its primary crystallization.
An analytical review of [13, 14] presents a critical analysis of the available information on the influence of external fields on the growth/synthesis of materials. The phenomenon of electrocrystallization is described in detail with emphasis on the peculiarities of electrocrystallization of metals as the most widely known use of electric fields in the crystallization of compounds. The features of electrocrystallization of oxides and salts are discussed in detail, it is shown that up to now a limited number of works on electrocrystallization of nonmetallic crystals in complex halide, and oxide systems are known. Moreover, the available works on this topic are fragmentary and aimed only at solving the utilitarian problem of obtaining crystals.
An attempt to explain changes in crystallizing slags under the effect of electric current was made by E. M. Kuznetsov. [15]. According to modern theory of crystallization [16] the nucleation rate depends exponentially on the rate of particle exchange between nucleate and liquid phase and on the probability of formation of crystallization centers.
2. Petrographic analysis of the mineral composition of oxide refractory materials
The effect of electromagnetic field on the crystallization process of oxide melts may be determined by elucidating the role of electromagnetic effects in the processes of crystal nucleation and growth, i.e. the effect on the behavior of the structural units of an oxide melt during crystallization. This, first, requires the establishment of the structure and components of oxide melts interacting with the external electromagnetic field.
Petrographic analysis of the mineral composition of the forsterite materials showed that they consist of prismatic and extended prismatic forsterite crystals (MgO⋅SiO2), magnesiferrite (MgO⋅Fe2O3) in the form of small irregularly shaped grains and rounded periclase grains (MgO) (Fig. 2(a)).
Fig. 2Microstructures of samples of a) forsterite, b) forsterite-chromite and c) corundum refractory materials
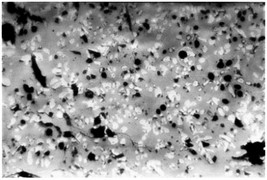
a)
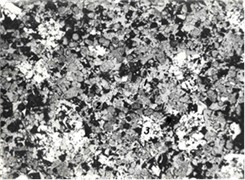
b)
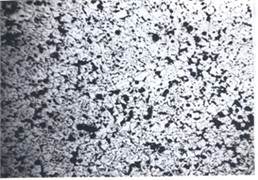
c)
The forsterite lattice (MgO⋅SiO2) refers to “isle” structures with separate tetrahedrons [SiO4-4], bound through ions Mg2+, forming irregular octahedral groups [MgO6]. Structural formula Mg[SiO4] [17, 18].
In forsterite-chromite materials, crystallises the following: forsterite (MgO⋅SiO2), periclase (MgO), spinelid (MgO⋅Fe2O3), as well as minor amounts (< 1 %) of glass phases and metal inclusions in the form of individual grains of 0.002-0.020 mm in size (Fig. 2(b)). Periclase crystallizes as grains (size 0.004-0.008 mm) of rounded and riziform. Its colour in ground joints varies from brown to transparent. In areas enriched with iron oxides (areas adjacent to unmelted chromite grains) periclase is dark brown, isotropic. In the forsterite matrix, outside contact with chromite, light brown and transparent grains of periclase occur. Forsterite crystallizes last in all zones of the block. It fills the gaps between the spinel and periclase crystals. The mineral is transparent, colourless in thin sections, with strong pleochroism.
Aluminium earth is more than 98 % aluminium oxide Al2O3 with inclusions of potassium, sodium, magnesium and other oxides. After calcination, aluminium earth mainly consists of a modification of α-Al2O3 (Fig. 2(с)).
3. Investigation of viscosity and electrical conductivity of melt oxide refractory materials
The main structure-sensitive characteristics of material melts are viscosity and electrical conductivity [19-24]. They are among the most important physical as well as technological characteristics of oxide refractory melts.
The temperature dependences of the viscosity and electrical conductivity of the forsterite, forsterite-chromite and partly corundum refractory materials in the range of (1800-2100) °С (Table 1).
Viscosity and conductivity studies were carried out on an electro-vibrating viscometer in molybdenum crucibles in a flow of inert gas in a Tammann furnace. The crystallisation temperatures were determined through the dependence of the logarithm of the viscosity on the inverse absolute temperature.
As table data shows, with increasing temperature dependences of forsterite and forsterite-chromite refractories practically degenerate, which indicates the absence of structural transformations in these melts. It was not possible to study the temperature dependences of the viscosity and electrical conductivity of corundum materials above 2100 °C due to the limited possibilities of the used Tammann furnace. Based on the literature, when overheating the melt Al2O3 over (50-100) °C the changes in the studied melt characteristics are also insignificant [25-27].
Table 1Viscosity and electrical conductivity of forsterite, forsterite-chromite and corundum refractory materials (Pa s / Sm/m)
No. | Refractory material | Temperature, С | ||||
1825 | 1850 | 1900 | 1950 | 2100 | ||
1 | Forsterite | 0,10/4,42 | 0,07/4,61 | 0,06/4,64 | 0,05/4,66 | |
2 | Forsterite-chromite | 0,12/4,52 | 0,07/3,93 | 0,06/4,10 | 0,06/4,13 | |
3 | Corundum | – | – | – | – | 0,06/- |
4. Analysis of the structure of melts of oxide refractory materials
According to modern understanding of the liquid state, when melting materials, liquids are produced whose structure and energy characteristics are close to those in the crystalline state [17,18]. Therefore, when describing the structure of oxide melts it is necessary to proceed from the crystal structure of mineral crystals. For this purpose the data obtained on viscosity and electrical conductivity were compared with mineral compositions (petrographic analysis) of samples used in experiments forsterite, forsterite-chromite and corundum refractories.
X-ray structure studies of the melt structure of the systems FeO-Fe2O3-CaO, FeO-Fe2O3-CaO-SiO2, FeO-Fe2O3-CaO-SiO2-Al2O3 indicate that at a concentration of FeO+Fe2O3 less than 20 % (molar) free ions in the melt Fe3+ are practically nonexistent, they are bound up in complexes of [Fe2O2-4] [17].
Proceeding from the above that in the melts studied the main structural units are anions [SiO4-4] and [Fe2O2-4]. The insignificant change in viscosity with increasing melt temperature up to 1950 0C indicates the absence of structural transformations in the melts of compositions in the temperature range considered. In view of the significant bonds within the complexes, they are less affected by thermal decomposition and retain their structure in this temperature range. The electrical conductivity of the melts is related to the presence of cations Mg2+.
In forsterite-chromite refractory melts, the main structural units are also anions [SiO4-4], [Fe2O2-4] and [Gr2O2-4]. Due to the considerable bonding within the complexes, they are also less affected by thermal decomposition and retain their structure in this temperature range. The electrical conductivity of the melts is also related to the presence of cations Mg2+.
With the melting of α-Al2O3 there are radical changes in structure and coordination. In the molten material the coordination number is 4.4. i.e. [AlO5-4] tetrahedrons are bonded to others in such a way that a structure similar to γ-Al2O3 and this structure does not change with temperature.
5. To the electromagnetic effect mechanism on the structure of oxide refractory materials
Based on the above analysis of various hypotheses and results of own studies, the authors for the first time propose the following explanation of the electromagnetic field mechanism on the crystallization process of oxide refractory melts, based on the periodic orientation effect of alternating electromagnetic field on the melt particles and accelerating the transport of particles to the crystallization front.
According to the cybotaxis theory [17, 18], the ordered structure of liquids is not limited to immediate neighbours, but extends to large volumes. They contain groups of particles different in size, where the order is preserved close to the order in the solid. The structure of cybotaxis is dynamic, inside they retain the solid structure and the outer layers lack strict symmetry.
When an external field is applied, the cybotaxis takes a certain orientation in the liquid volume according to its general charge. The field will force the particles to migrate in the volume, at the meeting of dissimilar particles the annihilation of charges will occur, accompanied with their enlargement and emission of excess of free energy. Thus, external energetic influence promotes intensification of the first stage of solid phase formation – development of centres of crystallization.
Crystal growth is judged by the rate of increase in its size, which is mainly determined by diffusion, i.e. by the conditions of particle supply to the crystal facets. Since growth of a crystal entirely depends on quantity of a substance coming to faces of that crystal, speed of growth of a crystal is determined by rate of diffusion. An electromagnetic field considerably increases the diffusion rate. Under the influence of the field the mobility of large formations and their transport to the surface of the crystal increases - this will increase the probability of settling of particles on its facets and thus the growth rate of the crystal.
As already established, forsterite and forsterite-chromite melts are composed of complex anions in the form of [SiO4-4], [Fe2O2-4] and [Gr2O2-4] and cations Mg2+. Aluminium earth is more than 98 % aluminium oxide Al2O3 with inclusions of potassium, sodium, magnesium, etc. Melting of α-Al2O3 results in radical changes in structure and coordination. In the molten material the coordination number is 4.4. i.e. [AlO5-4] tetrahedrons bond with others so that a γ-Al2O3-like structure is obtained and this structure does not change with temperature
When an external alternating electromagnetic field is applied to the crystallising melts of the studied forsterite, forsterite-chromite and corundum materials, their complex anions in the form of [SiO4-4], [Fe2O2-4], [Gr2O2-4] and [AlO5-4] begin to change their orientation and move periodically, following the direction of the field. Smaller and more mobile cations Mg2+ migrate intensively in the melt volume.
An increase in the collision probability of moving dissimilar particles with their subsequent enlargement contributes to an increase in the nucleation rate of crystallisation centers. Simultaneous formation of a large number of crystallisation centers leads to formation of fine crystalline structure of castings. The movement of structural units of the melt increases the number of particles transported and attached to the crystallization centers i.e. increases the rate of crystallization.
6. Conclusions
Comparison of the petrographic analysis of the mineral composition of the considered refractory compositions and the temperature dependences of the viscosity and electrical conductivity of their melts showed that the main structural units in the melts of forsterite materials are anions [SiO4-4] and [Fe2O2-4]; in forsterite-chromite melts - anions [SiO4-4], [Fe2O2-4] and [Gr2O2-4]; in corundum melts - anions [AlO5-4]. The electrical conductivity of the melts in the first two melts is related to the presence of cations in them Mg2+, and in the third – [Al3+].
The most probable mechanism of an alternating electromagnetic field effect on the structure of crystallising refractory castings is the orienting and intensifying migration action. When an external alternating electromagnetic field is applied, the complex anions in the melt[SiO4-4],[Fe2O2-4], [Gr2O2-4], [AlO5-4] begin to change their orientation and move periodically, following the direction of the field. Smaller and more mobile cations Mg2+migrate intensively in the melt volume. The increase in collision probability of moving dissimilar particles with their consequent enlargement contributes to an increased nucleation rate of crystallisation centres. Simultaneous formation of a large number of crystallization centres leads to formation of fine homogeneous structure of castings. The movement of structural units of the melt increases the number of particles transported and attached to the crystallization centres, i.e. contributes to an increase in the rate of crystallization.
References
-
X. Wence, C. Weili, J. Xiaolin, and Z. Xuefeng, “Effect of magnetic-field heat treatment on directional growth of magnetite in glass ceramics,” Materials Research Express, Vol. 6, No. 7, p. 075204, Apr. 2019, https://doi.org/10.1088/2053-1591/ab11ac
-
L. F. Alexander and N. Radacsi, “Application of electric fields for controlling crystallization,” CrystEngComm, Vol. 21, No. 34, pp. 5014–5031, Aug. 2019, https://doi.org/10.1039/c9ce00755e
-
S. V. Rudnev, “Modelling electromagnetic fields of structures of aluminum oxide materials for creating new treatment technologies,” (in Russian), in European Science and Technology: 2dn International scientific conference, pp. 346–350, 2012.
-
U. B. Ashimov, D. S. Satvaldiev, and Ye. A. Abdrakhmanov, “Study of plasmamelting refractory materials properties,” in 8th International Symposium on Plasma chemistry, pp. 1916–1919, 1987.
-
U. B. Ashimov and Ye. A. Abdrakhmanov., “Characterization of plasmamelted materials with electromagnetic mixing,” in 9th International Symposium on Plasma chemistry, pp. 792–795, 1989.
-
Ye. A. Abdrakhmanov, “Ordering by electromagnetic action of the structure and composition of crystallizing melts of oxide refractory compositions,” (in Russian), in International meeting “Order. Disorder and Properties”, pp. 5–8, 2010.
-
V. V. Slovikovsky and A. V. Gulyaeva, “Fused refractory oxides that increase the durability of linings of non-ferrous metallurgy units,” (in Russian), Journal of New Refractories, Vol. 10, pp. 3–8, 2016.
-
A. D. Razmyshlyaev and M. V. Ageeva, “On mechanism of weld metal structure refinement in arc welding under action of magnetic fields (Review),” (in Russian), Avtomatičeskaâ Svarka (Kiev), Vol. 2018, No. 3, pp. 29–33, Mar. 2018, https://doi.org/10.15407/as2018.03.05
-
V. P. Chernysh et al., Welding with Electromagnetic Stirring. (in Russian), Kyiv: Technique, 1983.
-
A. D. Razmyshlyaev and M. V. Ahieieva, “Features of arc surfacing process in a longitudinal magnetic field,” (in Russian), Applied Mechanics and Materials, Vol. 682, pp. 313–318, Oct. 2014, https://doi.org/10.4028/www.scientific.net/amm.682.313
-
A. D. Razmyshlyaev, P. A. Vydmysh, and M. V. Ageeva, Automatic Submerged Arc Welding with the Influence of an External Magnetic Field. (in Russian), Mariupol: PSTU Publishing House, 2017.
-
A. D. Razmyshlyaev and M. V. Ageeva, “The influence of a magnetic field on the crystallization of welds in arc welding,” (in Russian), Journal of Automatic Welding, Vol. 2019, No. 1, pp. 40–43, Jan. 2019, https://doi.org/10.15407/as2019.01.05
-
V. V. Kireev, “Solution-melt crystallization of oxide compounds under conditions of simultaneous exposure to electric and temperature fields,” (in Russian), Russian Center for Scientific Information, Jun. 2019.
-
D. A. Ksenofontov, “Investigation of the influence of an electric field on the growth of crystals by the solution-melt method,” (in Russian), 2010.
-
E. M. Kuznetsov, Crystallization of Blast-Furnace Slag Melts under the Action of Electric Current. (in Russian), Lipetsk: LPTI, 1983.
-
E. I. Marukovich, V. Y. Stetsenko, and A. V. Stetsenko, “Current state of metal melt crystallization theory. Foundry production and metallurgy,” (in Russian), Litiyo i Metallurgiya (Foundry Production and Metallurgy), No. 1, pp. 19–24, Mar. 2022, https://doi.org/10.21122/1683-6065-2022-1-19-24
-
N. A. Vatolin, Z. M. Kern, and V. L. Lisin, “X-ray study of the structure of silicate melts,” (in Russian), Structure and physico-chemical properties of metal and oxide melts, Sverdlovsk, 1986.
-
N. A. Vatolin and E. A. Pastukhov, Diffraction Studies of the Structure of High-Temperature Melts. (in Russian), Nauka, 1980.
-
N. L. Zhilo, I. S. Ostretsova, G. V. Charushnikova, and R. F. Pershina, “Viscosity and electrical conductivity of slags of the MgO – Al2O3 – SiO2 system with a high content of MgO,” (in Russian), Journal of Izvestiya VUZov. Ferrous Metallurgy, No. 4, pp. 35–40, 1982.
-
N. L. Zhilo et al., “Investigation of the physicochemical properties of slags of the MgO – SiO2 – Al2O3 system,” Journal of Metals, No. 4, pp. 25–31, 1980.
-
Kh. N. Kadarmetov, The Choice of Slags in the Smelting of Carbonaceous Ferrochromium and Ferrosilicochromium. (in Russian), 1982, pp. 66–75.
-
A. A. Zayakin, A. A. Akberdin, and V. I. Zhuchkov, “Physicochemical characteristics of oxide melts of MgO-Al2O3-SiO2-СаO-Cr2O3-FeO system,” (in Russian), Journal of Butlerov Communications, Vol. 48, No. 10, pp. 128–133, 2016.
-
M. Somerville, “Liquidus temperature and viscosity of melter slags,” in International Conference on Molten Slags, Fluxes and Salts, pp. 219–224, 2004.
-
J.-L. Li, A.-J. Xu, D.-F. He, Q.-X. Yang, and N.-Y. Tian, “Effect of FeO on the formation of spinel phases and chromium distribution in the CaO-SiO2-MgO-Al2O3-Cr2O3 system,” International Journal of Minerals, Metallurgy, and Materials, Vol. 20, No. 3, pp. 253–258, Mar. 2013, https://doi.org/10.1007/s12613-013-0720-9
-
V. P. Elyutin, B. S. Mitin, and Yu. A. Nagibin, “Electrical conductivity of liquid aluminum oxide,” (in Russian), Journal of Izvestiya Vuzov AN SSSR. A Series of Inorganic Materials, No. 7, pp. 880–881, 1971.
-
B. S. M. M. A. Maypax, Liquid Refractory Oxides. (in Russian), Metallurgy, 1979.
-
J. P. Coutures, J. C. Rifflet, P. Florian, and D. Massiot, “Termophisycal properties of liquid Aluminum oxide,” International Journal of High Temperatures and Refractories, Vol. 29, pp. 123–130, 1994.
About this article
The authors have not disclosed any funding.
The datasets generated during and/or analyzed during the current study are available from the corresponding author on reasonable request.
The authors declare that they have no conflict of interest.