Abstract
This study investigates the effect of gas supply pressure on power frequency amplitude, critical speed of rotation, whirl frequency and its corresponding power frequency, as well as on whip frequency and its corresponding power frequency on the basis of a high-speed turbine generator bearing-rotor system supported by a gas bearing and with changes in bearing gas supply pressure. Experimental results show that the optimization of gas supply pressure will reduce power frequency amplitude, improve critical speed, and delay the occurrence of whirl and whip. Under these conditions, the stability of the bearing-rotor system is improved, thus providing an experiment basis for the on-line monitoring and control of high-speed turbine generator operation.
1. Introduction
In the late 20th century, the establishment of power-distributed energy systems on the basis of the concept of the energy cascade utilization of heath as attracted considerable attention because of the changes in the demand for energy and power structures, as well as the changing requirements of carbon emissions and their effect on the environment worldwide [1]. High-speed turbine power generation technology is a key technology employed in distributed energy systems. In this technology, the stability of the rotor system comprising the compressor, turbine, and gas turbine coaxial structure bearing at a high speed is a key point [2-4].
Scholars around the world have studied gas bearing rotor system stability and thus achieved significant results. Luis San Andrs [5] used numerical and experimental methods to verify whether air pressure optimization can improve bearing stiffness, such that the critical speed of the rotor increases. Meanwhile, at a critical speed, the reasonable control of bearing pressure can effectively suppress vibration amplitude. Florin Dimofte [6] studied the effects of gas pressure on the stability of hydrodynamic-static hybrid bearings and drew a conclusion that an increase in initial bearing gas pressure can restrain and eliminate the low-frequency eddy of the rotor. In China, Yang Jinfu [7] proposed the coupling principle of frequency modulation and the engineering stability criterion. Chen Ce [8] studied the nonlinear dynamic behavior of a gas bearing-rotor system and improved the coupling principle of frequency modulation and the engineering stability criterion. Through experimental research, Han Dongjiang [9-10] drew a conclusion that a reasonable rise rate can control amplitude and that the use of a damping pad can effectively suppress the amplitude of the critical speed and the delayed low-frequency oscillations.
This study employs the tangential air inlet graphite alloy aerostatic bearing. To improve bearing stability, two O-rubber rings are placed on both ends of bearing. On the basis of the high-speed turbine generator bearing-rotor system supported by the gas bearing, we study the influence of the gas supply pressure on power frequency amplitude, critical speed of rotation, whirl frequency and its corresponding power frequency, as well as on whip frequency and it corresponding power frequency.
2. Experimental system and test plan of high-speed turbine generator
2.1. Test equipment and system
Fig. 1 shows a diagram of the test-bed and test system. The system includes four parts: test-bed body part, gas supply system, control system, and data acquisition and analysis system.
Fig. 1Test equipment and system
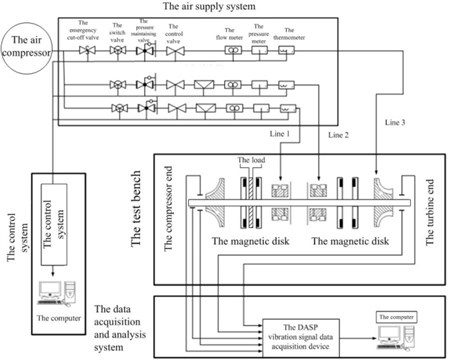
The bearing-rotor system in the test-bed is the gas lubrication bearing-rotor structure with a double gas thrust bearing surface arranged coaxially with a turbine wheel, compressor wheel, and four round disks (Fig. 2). The turbine wheel is driven by a high-pressure gas source (from the air compressor) and transfers the high-pressure gas source into a low-pressure gas by adiabatic expansion process. The compressor wheel is the driven wheel.
Fig. 2Graphite aerostatic radial and axial hybrid bearing
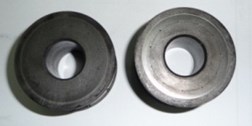
The gas supply system is divided in to the main gas line supply section (see Line 3 in Fig. 1) and bearing pneumatic supply section (Lines 1 and 2 in Fig. 1). The main gas line supply section provides high-pressure gas power for the body of the test-bed to rotate the bearing-rotor system by driving the turbine wheel. The bearing pneumatic supply section ensures a stable supply of high-pressure gas for hydrostatic gas bearing to support and lubricate the rotor.
The control system outputs signals artificially. The control valve in the gas supply system receives the signal and gives feedback to control the bearing gas pressure, drive the turbine wheel gas flow input, and finally control the bearing gas pressure and the rising rate of the rotor.
The data acquisition and analysis system consists of the electric eddy displacement sensor and data logger components. Two eddy current sensors are arranged orthogonally at the end of the shaft for measuring the vibration displacement from the horizontal and vertical directions. A key phase slot opens to the compressor terminal for measuring shaft speed and phase data.
The bearing is a graphite aerostatic radial and axial hybrid. The specific structure parameters are shown in Table 1. The material is graphite alloy characterized by high-temperature resistance and self-lubrication. Two O-rubber rings are placed on both ends of the bearing, and the rubber rings can increase system damping and improve system stability.
Table 1Bearing structure parameters
Parameters | Symbol / Unit | Numerical value |
Width | / mm | 50 |
Inner diameter | / mm | 25 |
Number of radial pores (each row) | / 1 | 16 |
Row number of radial pores | / 1 | 2 |
Space between each pore | / mm | 22.5 |
Number of axial pores | / 1 | 16 |
The hole diameter – orifice | mm | 0.4 mm |
2.2. The experimental scheme
As shown in Table 2, three sets of experiments were conducted to study the effect of bearing gas pressure on the vibration characteristics of rotor system. Two bearing tracheal roads were used: 1# and 2#. The 1# bearing tracheal path supplies gas to the bearing of turbine end, whereas 2# supplies gas to the bearing of the compressor end.
Table 2Experimental scheme of bearing gas supply pressure
Experimental number | Bearing gas supply pressure | Average pressure | |
The first group test | 1#–0.70 MPa | 2#–0.70 MPa | 0.70 MPa |
The second group test | 1#–0.75 MPa | 2#–0.75 MPa | 0.75 MPa |
The third group test | 1#–0.78 MPa | 2#–0.78 MPa | 0.78 MPa |
3. Acc test analysis of high-speed turbine generator
3.1. Overall description of three-dimensional spectra
Figs. 3 to 5 show the three-dimensional time-frequency-amplitude spectra of the speed-up process. The abscissa is shown as frequency, the ordinate is shown as time, and the depth of color represents the amplitude of frequency. A brighter color indicates larger amplitude. The graph indicates that the three sets of tests showed half speed vortex and low frequency oscillation. With the increase in air pressure bearings, the frequency of whirl, the frequency of whip, and their corresponding power frequency speed were delayed.
Fig. 3Three-dimensional spectra of the first group test
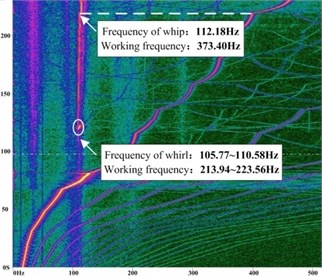
Fig. 4Three-dimensional spectra of the second group test
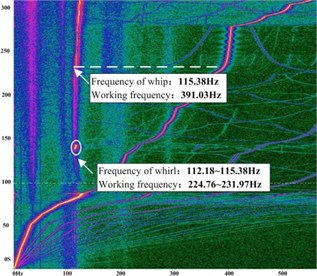
3.2. Amplitude of power frequency
Fig. 6 shows that the curve that power frequency amplitude changes with bearing gas supply pressure. When the rotor is through the critical speed, amplitude decreases with the optimization of the bearing supply pressure. However, the change trend of amplitude is consistent. Therefore, improving the bearing supply pressure can enhance the security and stability of the bearing rotor system.
Fig. 5Three-dimensional spectra of the third group test
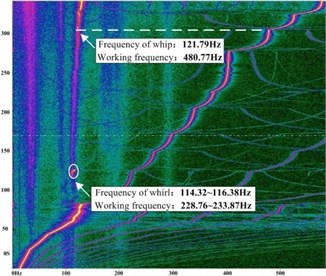
Fig. 6Power frequency amplitude changes with bearing gas supply pressure
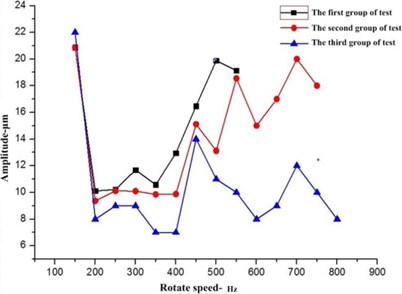
Fig. 7Critical speed changes with bearing gas supply pressure
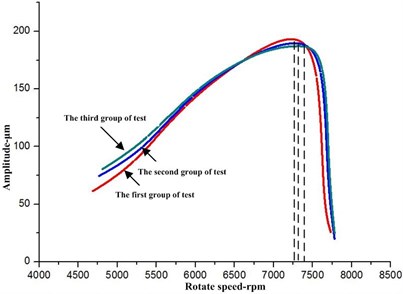
3.3. Critical speed of rotation
The resonance frequency of the bearing rotor system is determined by stiffness, whereas the amplitude is determined by damping. With the increase in gas pressure, gas film stiffness and damping increase. Therefore, the increase in bearing gas pressure delays the critical speed and reduced the amplitude slightly, as shown in Fig. 7.
3.4. Whirl and whip
Figs. 8 and 9 show the relationship curve among the frequency of whirl and whip, their corresponding power frequency, and bearing gas supply pressure. The starting frequency of half-speed vortex can be seen from the curve, and the corresponding power frequency increases with the increase in bearing pressure. The half-speed whirl frequency of the three sets of tests increased from 105.77 Hz to 114.32 Hz. The corresponding frequency increased from 213.94 Hz to 228.76 Hz. Meanwhile, the starting frequency of low-frequency oscillations and corresponding power frequency increased with the increase in bearing pressure. The whip frequency of the three sets of tests increased from 112.18 Hz to 121.79 Hz. The corresponding frequency increased from 373.40 Hz to 480.77 Hz.
The typical bifurcation diagrams and axis trace of the whirl and whip in the three sets of tests are shown in Figs. 10 and 11. The axis trace of whirl is shown as a typical “inside eight”. The axis trace of whip runs in cycle four, and the corresponding bifurcation diagram is divergent but not infinite. This condition indicates that the amplitude of the chaos boundary is bounded.
Therefore, a higher bearing gas supply pressure can effectively control and restrain the occurrence of whirl and whip, delay bearing-rotor system instability, and improve system stability.
Fig. 8Frequency of whirl and whip with changes in bearing gas supply pressure
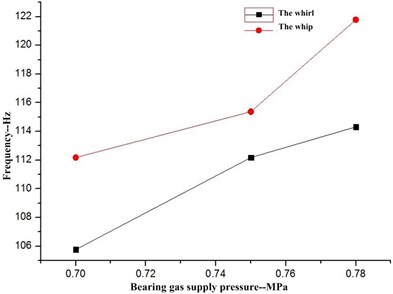
Fig. 9Working frequency with changes in bearing gas supply pressure
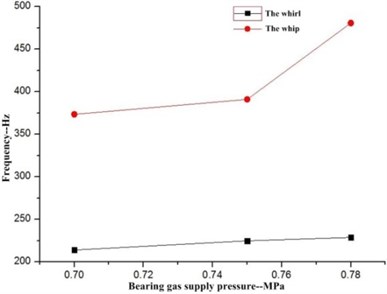
Fig. 10Bifurcation diagrams and axis trace of the whirl phenomenon
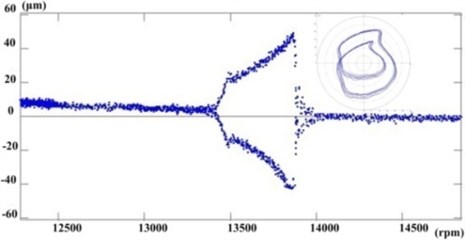
Fig. 11Bifurcation diagrams and axis trace of the whip phenomenon
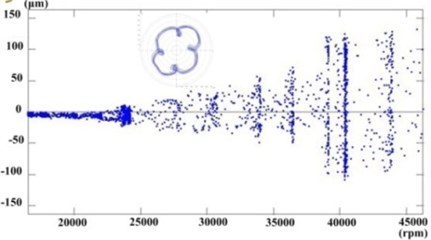
4. Conclusions
1) To improve the bearing gas pressure from 0.7 MPa to 0.78 MPa, the critical speed can be reduced from 7,262 rpm to 7,330 rpm, and the amplitude of critical speed can be reduced from 204.5 μm to 192.2 μm.
2) The optimization of bearing gas pressure can reduce the power frequency amplitude of the whole acc process.
3) To improve the bearing gas pressure from 0.7 MPa to 0.78 MPa, the frequency of whirl can be increased from 105.77 Hz to 114.32 Hz. The corresponding working frequency increased from 213.94 Hz to 228.76 Hz. Meanwhile, the frequency of whip increased from 112.18 Hz to 121.79 Hz, and the corresponding working frequency increased from 373.40 Hz to 480.77 Hz.
4) In this study, the conclusion can provide an experimental basis for the online monitoring and control of high-speed turbine generator operations.
References
-
Wang Kai, Huang Baohua, Tian Yunfeng Feasibility study of micro gas turbine in distributed energy supply. North China Electric Power, Vol. 5, 2011, p. 1-5, 15, (in Chinese).
-
Yang Jinfu, Yang Chenbo, Chen Ce, et al. Research on sliding bearings and rotor system stability. Journal of Aerospace Power, Vol. 23, Issue 8, 2008, p. 1420-1426, (in Chinese).
-
Yang Jinfu Study on stability of fluid dynamic lubricates and bearing rotor system. North China Electric Power University, Beijing, 2006, (in Chinese).
-
Chen Ce, Yang Jinfu, Nie Chaoqun Experimental research on nonlinear dynamic characteristic of gas lubrication bearings and high speed rotors. Journal of Aerospace Power, Vol. 23, Issue 8, 2008, p. 1413-1419, (in Chinese).
-
Luis San Andrs, Keun Ryu Hybrid gas bearings with controlled supply pressure to eliminate rotor vibrations while crossing system critical speeds. Journal of Engineering, Vol. 130, Issue 6, 2008.
-
Florin Dimofte, SorinCioc, et al. On the influence of the supply pressure on the dynamic stability of hybrid gas journal wave bearings. World Tribology Congress III, USA, Washington, 2005, p. 12-16.
-
Yang Jinfu, Liu Zhansheng, Yu Daren, et al. Research on nonlinear oil film force and its stability of journal bearing. Power Engineering, Vol. 24, Issue 4, 2004, p. 501-504, 597, (in Chinese).
-
Chen Ce Research on rotor-bearing system nonlinear dynamics behaviors, fluid-solid interaction and its frequency modulations. Beijing, Institute of Engineering Thermophysics, Chinese Academy of Sciences, 2008, (in Chinese).
-
Han Dongjiang, Yang Jinfu, Geng Jiamin, et al. Vibration characteristics of gas bearing-rotor system of high-speed permanent magnet machine. Journal of Aerospace Power, Vol. 28, Issue 8, 2013, p. 1791-1796, (in Chinese).
-
Han Dongjiang, Yang Jinfu, Zhao Chen, et al. An experimental study on vibration characteristics for gas bearing-rotor system. Journal of Vibration Engineering, Vol. 25, Issue 6, 2012, p. 680-685, (in Chinese).