Abstract
As separated platform subway station structures are close to bridge piles, their analysis under static and seismic loading is required. An artificial earthquake record, generated by using Kanai-Tajimi model and trigonometric series superposition method, is used to analyze the seismic performance of the subway station structure. Different construction sequences of bridge piles and subway stations are considered in the analysis of structural seismic performance. Irrespective of the type of analysis, most of the horizontal and the vertical stresses of the subway station with existing bridge piles show a decreasing trend as compared to those without bridge piles. The stresses associated with post-constructed bridge piles increase significantly as compared to those without piles, especially in areas of stress concentrations. A parametric analysis of the distance between the subway station and the bridge pile is also conducted. The percentage increase in horizontal and vertical stress of the subway station, with post-construction pile, gradually decreases with increasing distance. However, in case of existing piles the percentage increase in stress, as a function of distance, has an inflection point.
1. Introduction
The growing trend of construction of underground structures and their vulnerability to damage during earthquake has emphasized the importance of earthquake-resistant characteristics of various types of such structures. In the 1995 Kobe earthquake, significant damage occurred to the subway system in Kobe, Japan, including the collapse of the Daikai subway station [1]. In the 1999 Taiwan earthquake, damage to the mountain tunnels in central Taiwan was considerable [2]. During the 1999 Kocaeli earthquake in Turkey, a highway tunnel severely collapsed [3]. Additionally, damage to large underground structures was reported in other earthquakes, such as in the 1976 Tangshan earthquake in China, the 1989 Loma Prieta earthquake in the USA [4], the 2004 Baladeh Iran earthquake [5] and the 2008 Wenchuan earthquake in China.
As underground reinforced concrete (RC) structures interact with the surrounding soil medium, the behavior of the interfacial zone between the RC structure and the surrounding medium of the underground RC structure should be considered for accurate seismic analysis. Nam developed an elasto-plastic interface element which considers thickness of the interface for simulating the seismic behavior of underground RC structures, and the effect of the interfacial behavior between the structure and the surrounding soil [6]. Pakbaz analyzed the interaction between the ground and the tunnel lining during an earthquake excitation. The results showed that the effects of an earthquake on tunnel-ground interaction depend on various parameters, like peak acceleration, intensity and duration of the earthquake and relative rigidity between the tunnel and the ground [5]. Lin and Chen studied the effect of various factors such as buried depth, condition of soil layers and structure style on the earthquake-resistant capacity of underground structures [7]. It was demonstrated that the buried depth had great effect on the seismic responses of the underground structure, and the earthquake-resistant capacity of the structure could be improved greatly if the soil layers and structure type were appropriately selected. The observations from recent earthquakes have shown that the response of the foundation and soil can greatly influence the overall structural response. There are several cases of severe damage to structures due to soil-structure interaction in past earthquakes. The Beijing Hujialou metro station was designed to be adjacent to the Jingguang Bridge. A FEM analysis was carried out considering PBA (pile-beam-arch) construction method. The analysis indicated that PBA method used in the construction of the metro station was reasonable [8]. The results of a study conducted on short-piled overpass foundation in the vicinity of metro construction indicated that settlement of pile foundation can be effectively controlled by underpinning [9].
The separated platform subway station were created due interference of construction of subway stations with underground urban facility. This type of under-bridge subway station is often very close to the bridge piles. The Guomao Station is an interchange station between Line 1 and Line 10. It is a typical separated platform subway station. Because the structure is close to the bridge piles, it is necessary to analyze the seismic performances of the separated platform subway station structure to ensure the structural safety. In this study, the influence of bridge piles on the seismic response of the subway station structure is investigated.
2. Generation of artificial earthquake records
The stochastic field model is often used to simulate seismic ground motion. The time process of the seismic ground motion is expressed as follows:
where is a definite time envelope function; is the stationary stochastic process and is generated by a trigonometric series superposition method:
where ; the initial phase is distributed random variables generated by uniformly distributed random numbers in the region [0-2].
Fig. 1The time-history curve of artificial earthquake
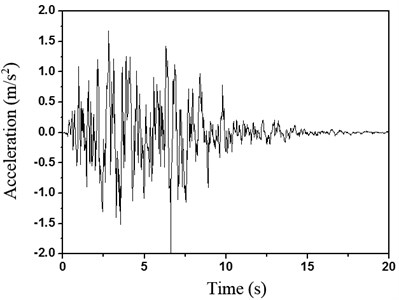
Based on Kanai’s investigation regarding the frequency content of different earthquake records, Tajimi proposed the spectral density function of the strong ground motion with a distinct dominant frequency as follows [10]:
where and are the site dominant damping coefficient and frequency, respectively; and is the constant power spectral intensity of the bed rock excitation. In practice, these parameters need to be estimated from local earthquake records and/or site geological features.
The material damping matrix is often assumed to be of the Rayleigh form. Rayleigh damping is proportional to mass and stiffness matrices [11].
The artificial earthquake ground acceleration of the Guomao Station with a maximum ground acceleration of 0.2 g based on the Kanai-Tajimi power spectral density function is shown in Fig. 1.
3. Numerical modeling
To study the impact of adjacent piles on the performance of the Guomao Station, a two-dimensional FEM with ABAQUS of the structure with piles has been built, as shown in Fig. 2.
Fig. 2A two-dimensional FEM of the structure with piles
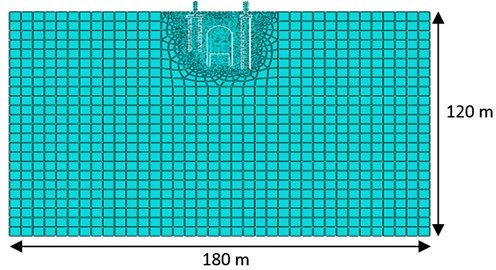
The Guomao Station arch crown is located at a depth of 7.7 m below the ground’s surface. The left and right piles are 1.2 m in width and 18 m, 27 m in height, respectively (see Fig. 3). To eliminate the boundary effect on the dynamic responses of the structure, the width and height of the soil are set as 180 m and 120 m, respectively.
Fig. 3The bridge piles and the subway station
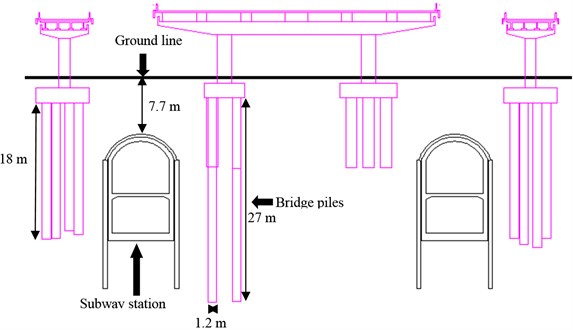
The material parameters in the current study are based on subway construction reports. The Mohr-Coulomb plastic model is used as a soil constitutive model, and the material parameters can be observed in Table 1.
The parameters of the station are as follows: the density is 2551 kg/m3, the elastic modulus is 30000 MPa and the Poisson’s ratio is 0.167. The structure and soil are modeled by plane strain elements. The bottom grid is pinned and horizontal displacement of lateral boundaries is restrained. Three conditions without piles, with existing piles (piles exist, then construct subway stations) and with post-constructed piles (subway stations exist before constructing piles) are considered.
Table 1Material parameters of the soil
Elastic modulus (MPa) | Poisson’s ratio | Density (kg/m3) | Friction angle (°) | Dilation angle (°) | Cohesion yield stress (Pa) |
27.3 | 0.35 | 1600 | 25.5 | 25.5 | 34700 |
4. Static and dynamic analysis of subway station structure
The piles adjacent to the subway station will inevitably affect the behavior of the subway station due to the pile-soil-structure interaction, resulting in changes in the stress conditions of the subway station.
4.1. Static analysis
According to the static analysis, most of the horizontal stress with existing piles tends to be smaller compared to that without piles in stress concentrated areas. As observed in Fig. 4, the percentage decrease in horizontal stress is largest at the outer wall corner, which is 16.9 %. Concurrently, the vertical stress with existing piles has a decreasing trend. The inner wall corner where has the largest vertical stress value reveals a significant stress reduction of 8.7 %, as shown in Fig. 4. There are two main causes for the changes in stress with existing piles. First, bridge piles can resist a portion of the soil pressure, thus reduce the structural stress. Most nodal stresses of the structure are affected for this reason. The increasing trend of horizontal stresses around the arch is due to the action of the piles blocking the outward thrust of the arch crown.
As for the stress with post-constructed piles, both the horizontal and vertical stresses have a tendency to increase. The horizontal stress of the outer wall corner and the vertical stress of the inner arch foot are increased by 24.6 % and 33.6 %, respectively. Because bridge piles are constructed after the subway station, the added gravity load of the bridge structure will cause the vertical stress to increase. Similar to existing piles effect, the outward thrust of the bridge arch crown will be restraint by piles resulting in an increase of the horizontal stresses.
Fig. 4Stress percentage increase of existing and post-constructed piles compared to that without piles. Node number: 1 – Upper station arch crown center, 2 – Lower arch crown center, 3 – Inner arch foot, 4 – Outer arch foot, 5 – Upper diaphragm slab center, 6 – Lower diaphragm slab center, 7 – Inner diaphragm slab and side wall border, 8 – Outer diaphragm slab and side wall border, 9 – Upper floor center, 10 – Lower floor center, 11 – Inner wall corner, 12 – Outer wall corner
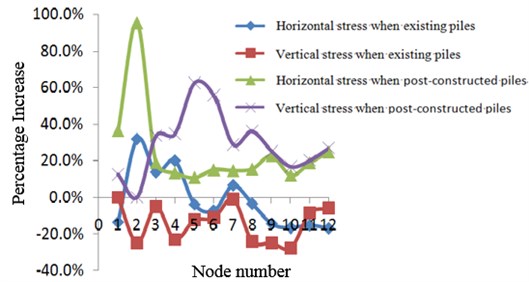
4.2. Dynamic analysis
Compared to the static analysis, most of nodal maximum stresses under earthquake excitation increase. Table 2 shows the significant differences. Therefore, it’s necessary to find out the effect of bridge piles on station under seismic excitation.
Table 2Stress percentage increase under earthquake excitation compared to static analysis
Without piles | Existing piles | Post-constructed piles | ||
Horizontal stress | Inner arch foot | 16.2 % | 16.7 % | 15.9% |
Outer arch foot | 13.3 % | 11.1 % | 11.8 % | |
Vertical stress | Outer arch foot | 23.1 % | 35.0 % | 20.0 % |
Inner diaphragm slab and side wall border | 23.1 % | 26.2 % | 20.1 % | |
Inner wall corner | 9.6 % | 12.9 % | 9.8 % |
It can be observed in Fig. 5 that the results under the dynamic analysis have a similar trend compared to that under the static analysis except for the vertical stress percentage increase of upper and lower diaphragm slab center. The vertical stresses with existing piles show a decreasing trend under the earthquake excitation. While the vertical stresses with post-constructed piles increase, the inner arch foot, the inner diaphragm slab and side wall border, and the inner wall corner increase by 29.7 %, 25.8 % and 20.3 %, respectively; all these area have relatively significant stress values.
Conversely, most of the horizontal stresses with existing piles show a decreasing trend compared to that without piles; however, the horizontal stresses in the lower arch crown center, inner arch foot, outer arch foot and inner diaphragm slab and side wall border increased by 31.7 %, 14.0 %, 17.6 % and 6.3 %, respectively. Meanwhile, the horizontal stresses with post-constructed piles increased, led by the lower and upper arch crown center, by 95.1 % and 36.4 %, respectively.
Fig. 5Stress percentage increase of existing and post-constructed piles compared to that without piles under earthquake excitation. Node number: 1 – Upper station arch crown center, 2 – Lower arch crown center, 3 – Inner arch foot, 4 – Outer arch foot, 5 – Upper diaphragm slab center, 6 – Lower diaphragm slab center, 7 – Inner diaphragm slab and side wall border, 8 – Outer diaphragm slab and side wall border, 9 – Upper floor center, 10 – Lower floor center, 11 – Inner wall corner, 12 – Outer wall corner
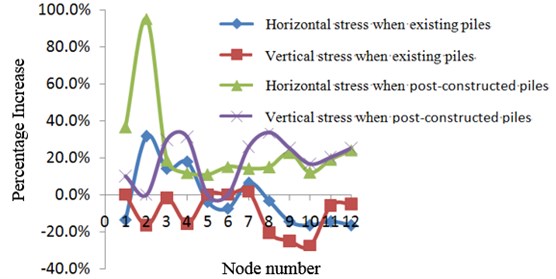
Overall, there is a significant increasing trend in subway stations with post-constructed piles irrespective of being under static or dynamic analyses. Therefore, it is necessary to consider the influence of piles on subway stations under seismic analysis, especially in stress concentrated areas, such as wall corners, arch feet, diaphragms, side wall borders, etc. As for the station with existing piles, increased stress areas, such as the lower arch crown center, the inner arch foot, the outer arch foot, the inner diaphragm slab and the side wall border, should be given more attention.
5. Parameter analysis
There are many factors that can affect the influence of bridge piles on the subway station, such as the distance between the subway station and the bridge pile. Therefore, parameter analysis was conducted to find out the influence of the distance between the station and bridge pile on the seismic performance of the subway station.
5.1. Post-constructed piles
According to the Guomao Station construction reports, the horizontal distance between the subway station and the nearest bridge pile is 2.8 m. During the finite element analysis, six different distances are set: 2.8 m, 12.8 m, 22.8 m, 32.8 m, 42.8 m and 52.8 m, while other factors remain unchanged. After numerical simulation, the stress of subway station under different distance was compared with that without piles. Fig. 6 and Fig. 7 show the relationship between the percentage increase of the subway station stress and different distance.
Fig. 6The relationship between the percentage of horizontal stress increase and the distance
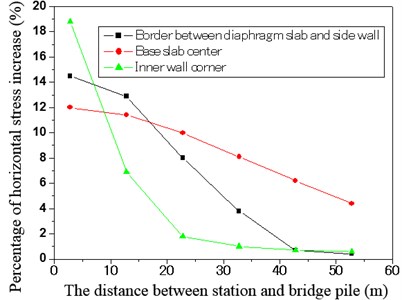
Fig. 7The relationship between the percentage of vertical stress increase and the distance
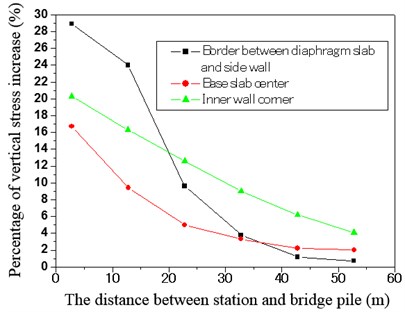
It can be seen from Fig. 6 and Fig. 7 that the percentage increase in horizontal and vertical stress gradually decrease with increase in distance between the subway station and bridge piles. As the distance increase, the constraint of bridge piles on station is decreasing and the subway station stress is also decreasing. The percentage increase is less than 5 % when the distance between the subway station and the bridge piles exceeds 50 m and thus the effects of bridge piles on seismic response of subway station can be ignored.
5.2. Existing piles
As for the existing piles situation, six different distances are set: 2.8 m, 12.8 m, 17.8 m, 22.8 m, 32.8 m and 42.8 m. Then the seismic performance of station was analyzed using the same method as that with post-constructed piles.
In Fig. 8, the percentage of stress increase has an inflection point, that is, the percentage of stress increase grows first and then declines. It is due to the interaction of two main factors which are the soil pressure between the subway station and bridge piles and the constraint of the bridge piles on the subway station. When the distance is small, as the distance increase, the bridge piles prevent the soil pressure decrease, so the structural stress increases. As the distance is large, the constraint of bridge piles on station is decreasing and the structural stress is also decreasing. The percentage of station stress increase is also less than 5 % when the distance between the subway station and the bridge piles exceeds 50 m. Thus, the effects of the bridge piles on the subway station seismic responses can also be ignored.
Fig. 8The relationship between the percentage of stress increase and the distance
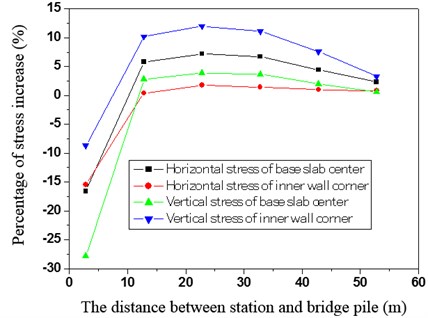
6. Conclusions
Piles adjacent to the subway station change the stress boundary conditions of the subway station and the stress of the subway station. Moreover, different construction sequences of bridge piles and subway stations have different influences on the stresses of the subway station. A numerical analysis is conducted to study the static and dynamic response influence of bridge piles on the subway station. The following conclusions can be drawn from the current study:
1) When bridge piles is existing, the subway station stresses show a decreasing trend compared to that without piles. The subway station stresses with post-constructed bridge piles increase significantly as compared to that without piles.
2) Different construction sequences of bridge piles and subway stations have different effects on structural dynamic behavior. For post-constructed piles, the percentage of station horizontal and vertical stress increase gradually decrease with the growing distance. However, for the subway station with existing bridge piles, the percentage of station horizontal and vertical stress increase increases sharply first, then gradually decrease with the growing distance.
References
-
An X. H., Ashraf, A., Shawky K. M. The collapse mechanism of a subway station during the Great Hanshin earthquake. Cement and Concrete Composites, Vol. 19, Issue 3, 1997, p. 241-257.
-
Wang W. L., Wang T. T., Su J. J., et al. Assessment of damage in mountain tunnels due to the Taiwan Chi-Chi Earthquake. Tunnelling and Underground Space Technology, Vol. 16, Issue 3, 2001, p. 133-150.
-
Hashash Y. M. A., Hook J. J., Schmidt B., et al. Seismic design and analysis of underground structures. Tunnelling and Underground Space Technology, Vol. 16, Issue 4, 2001, p. 247-293.
-
Azadi M., Hosseini S. M., Mir M. Analyses of the effect of seismic behavior of shallow tunnels in liquefiable grounds. Tunnelling and Underground Space Technology, Vol. 25, Issue 5, 2010, p. 543-552.
-
Pakbaz M. C., Yareevand A. 2-D analysis of circular tunnel against earthquake loading. Tunnelling and Underground Space Technology, Vol. 20, Issue 5, 2005, p. 411-417.
-
Nam S. H., Song H. W., Byun K. J., et al. Seismic analysis of underground reinforced concrete structures considering elasto-plastic interface element with thickness. Engineering Structures, Vol. 28, Issue 8, 2006, p. 1122-1131.
-
Lin L. M., Chen J. Y. Analysis of earthquake-resistant capacity of shallow-buried subway station structures in soft soil. Journal of Disaster Prevention and Mitigation Engineering, Vol. 26, Issue 3, 2006, p. 268-273, (in Chinese).
-
Li Z. P., Zhu Y. S. Research on construction method of metro station adjacent to bridge piles and reinforcement measures for bridge piles. Chinese Journal of Rock Mechanics and Engineering, Vol. 27, 2008, p. 3132-3139, (in Chinese).
-
Wu B., Liu W. N., Suo X. M., et al. A study on the reinforcement of a short-piled overpass foundation in the vicinity of metro construction. China Civil Engineering Journal, Vol. 39, Issue 7, 2006, p. 99-103, (in Chinese).
-
Rofooei F. R., Mobarake A., Ahmadi G. Generation of artificial earthquake records with a nonstationary Kanai-Tajimi model. Engineering Structures, Vol. 23, Issue 7, 2001, p. 827-837.
-
Erduran E. Evaluation of Rayleigh damping and its influence on engineering demand parameter estimates. Earthquake Engineering and Structural Dynamics, Vol. 41, Issue 14, 2012, p. 1905-1919.
About this article
This research is financially supported by the National Natural Science Foundation of China under Grant No. 51008011, China Scholarship Council under Grant No. 201206025022, and National Key Technology R&D Program of the Ministry of Science and Technology of the People’s Republic of China under Grant No. 2006BAJ27B02.