Abstract
The objective of this study is to investigate the symptoms, types, etiology, and assessment methods of motion sickness in autonomous vehicles in order to gain a comprehensive understanding of its occurrence mechanism and emphasize the significance of enhancing autonomous vehicle algorithms for improved ride comfort. Thus, this paper provides a synthesis and discussion of various theories while exploring strategies for mitigating motion sickness from three perspectives: passengers, vehicles, and external equipment. Firstly, it summarizes the clinical manifestations and classification of motion sickness while conducting an in-depth analysis of associated factors. Secondly, it evaluates different approaches for quantitatively measuring the severity and extent of motion sickness. Subsequently, it analyzes the reasons behind increased motion sickness caused by autonomous vehicles and emphasizes the importance of algorithmic improvements to enhance travel comfort. Finally, mitigation strategies are proposed considering passengers' needs as well as advancements in accurate motion prediction models and optimization techniques for autonomous planning and control algorithms that can effectively reduce the risk of motion sickness. As application scenarios for autonomous technology continue to expand, meeting user requirements while ensuring safety has become a benchmark for assessing technical proficiency. Therefore, promoting unmanned travel services necessitates a thorough analysis of existing issues related to autonomous technology along with prioritizing algorithm design enhancements through effective means to achieve an enhanced user experience.
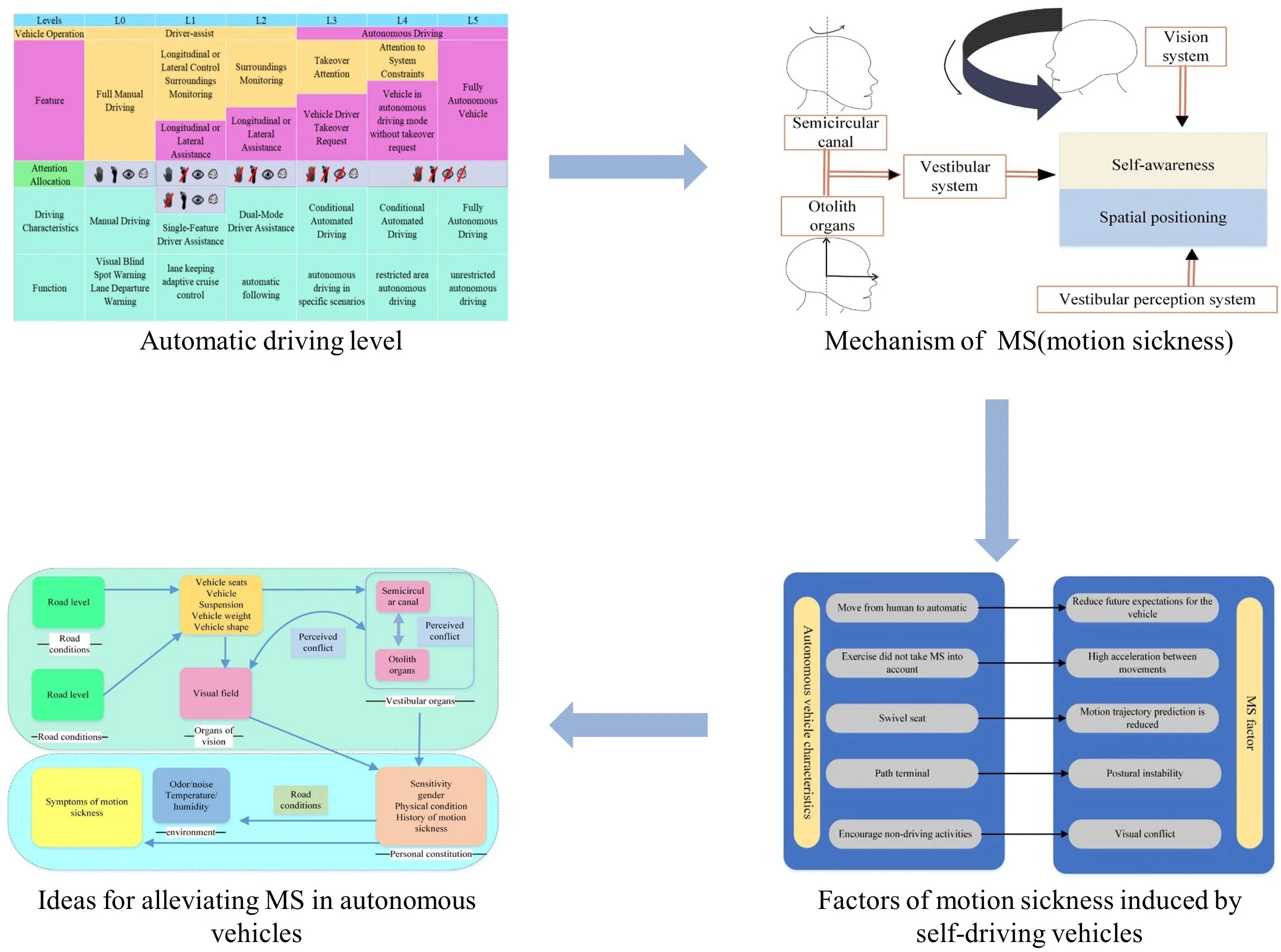
Highlights
- First of all, the classification of automatic driving is introduced, with special attention to the vehicle reaching the level of automatic driving above LV3, because high levels of automatic driving may cause motion sickness.
- Then the paper introduces the motion sickness disease causes, analyzes the conditions of the cause of motion sickness disease and characteristics.
- According to the characteristics of automatic driving vehicle is analyzed, and compared with the characteristics of motion sickness disease produces, deduce the self-driving cars may induce halo movement disorder.
- From two angles of vehicles and passengers may alleviate the method of motion sickness disease is discussed, and put forward the solving motion sickness disease challenges faced, and provided some promising research directions.
1. Introduction
The emergence of autonomous driving technology has positioned it as one of the most pivotal advancements in the automotive industry [1]. However, the proliferation of autonomous vehicles has raised concerns regarding an increased susceptibility to motion sickness [2]. According to the American Society of Automotive Engineers, autonomous driving vehicles are classified from L0 to L5 based on their level of intelligence [3], as shown in Fig. 1. The rapid development of autonomous driving necessitates novel approaches to enhance vehicle ride comfort, particularly considering that in highly automated vehicles of the future, human drivers will be transformed into passengers who can engage in various activities except for driving. While being relieved from demanding "driving tasks," especially in congested urban traffic, would significantly reduce human workload, assuming a passenger role may present challenges related to ride comfort, particularly for individuals prone to motion sickness. In recent years, there has been significant growth in the global market for motion sickness drug treatments. According to data released by QYResearch Medical and Health Research Center, anticholinergic motion sickness drugs and antihistamines have exhibited a compound annual growth rate exceeding 2 % between 2019 and 2024. This indicates an increasing number of patients suffering from motion sickness [4].
Fig. 1Autonomous driving level diagram
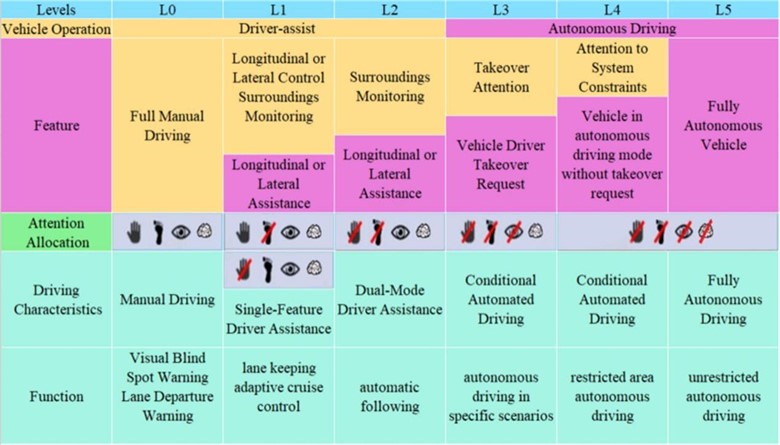
By integrating multiple sensors [5] and optimization algorithms [6], autonomous driving technology can effectively detect obstacles and traffic signal signs [7], thereby enhancing driving safety in adverse weather conditions and reducing the risk of accidents. Moreover, autonomous driving can also mitigate accidents caused by human error, such as fatigue [8], distraction, improper operation, and other factors, further bolstering the reliability and safety of driving [9]. Additionally, autonomous driving not only improves the safety of driving but also enhances driver comfort and convenience [10]. The driver no longer needs to focus solely on the task at hand but can engage in other activities like reading, watching videos, or interacting with passengers, making the overall driving experience more relaxed and enjoyable [11]. Furthermore, autonomous driving can provide transportation services for individuals who lack the ability to drive (e.g., elderly people, children, or disabled individuals) [12], and optimize travel routes for improved efficiency while reducing traffic congestion and commuting time. This ultimately leads to reduced energy consumption and emissions as well [13]. Autonomous driving represents one of the most significant trends in the automotive industry as it not only enhances road safety but also offers convenient and environmentally friendly means of transportation. However, transitioning from a driver to a passenger in an automated vehicle eliminates our ability to predict its trajectory accurately. Consequently, this may lead to increased occurrences of motion sickness due to uncertainties surrounding path planning effectiveness or smoothness during automated drives.
In order to ensure a safe arrival at the destination, ride comfort is the most crucial criterion for passengers to evaluate the merits of autonomous mobility services. Currently, car companies primarily focus on vehicle route planning, emphasizing efficiency and safety from point A to point B, without addressing passenger movement concerns. It is worth noting that companies like Apple Inc. and OPPO have started tackling motion sickness issues through mobile phones, but few have approached it from a vehicular perspective. Therefore, future research should consider both path planning and motion sickness in passengers. Investigating motion sickness in autonomous vehicles holds significant importance in promoting autonomous travel services and enhancing service levels of such vehicles. This paper reviews and discusses symptoms, types, etiology, and evaluation methods of motion sickness while exploring approaches to alleviate it from passenger-centric perspectives as well as through vehicle and external equipment considerations. Finally, this paper highlights existing research limitations and outlines prospective directions for future studies on motion sickness in autonomous vehicles.
The article is organized as follows. Chapter 2 introduces the symptoms and types of motion sickness. The factors affecting motion sickness are introduced in Chapter 3. Chapter 4 introduces how to detect and evaluate motion sickness. In Chapter 5, we introduce how to relieve motion sickness from three aspects: external equipment, personal, and vehicle. The conclusion is given at the end of the article.
2. Mechanism of motion sickness in autonomous vehicles
2.1. Mechanism of motion sickness
Motion sickness was first documented over 2,000 years ago by the Greek physician Hippocrates, who observed that “motion disturbs the body when sailing at sea” [14]. This condition can manifest in various environments such as land, sea, air, space, and simulators. Common symptoms of motion sickness encompass nausea, vomiting, sweating, fatigue, disorientation, dizziness, and loss of coordination (Table 1). Humans rely on multiple sensory systems for spatial orientation [15], including proprioceptive input from limbs and torso movements; vestibular input from the perception of balance through the vestibular system; and visual input for establishing a visual framework [16]. These three interdependent sensory systems coordinate to form a spatial reference system. Disruption of this system can lead to discomfort. Currently regarded as a natural response to an unnatural environment, motion sickness is commonly explained by theories such as sensory conflict theory [17], evolutionary theory [18], and postural instability theory [19].
Sensory conflict theory: According to the sensory conflict theory, motion sickness arises from a discrepancy between the internal sensory system and the external visual system. When there is an inconsistency between bodily sensations of movement and visual perception, it can trigger symptoms such as nausea, dizziness, and vomiting. For instance, in a boat or car, motion sickness occurs when the internal sensory system detects body movement while the external visual system fails to recognize this movement, resulting in a conflict between these two systems.
Evolutionary theory: The evolutionary theory posits that motion sickness is a natural response developed through human evolution. This theory suggests that symptoms of motion sickness served as an adaptive genetic pattern for our ancestors to avoid ingesting potentially harmful substances. The human inner ear and balance system are sensitive to detecting and responding to potential toxins, leading to symptoms of motion sickness that compel individuals to steer clear of hazardous situations.
Postural instability theory: Postural instability theory states that prolonged movements cause postural instability which leads to motion sickness. For example, after extended periods of travel by car, boat, or plane, the body's balance system gradually adapts itself to continuous movement; however, when this movement ceases abruptly, the balance system remains in an adapted state causing symptoms of motion sickness. This theory explains motion sickness as maladjustment and imbalance within the postural system.
Table 1Table of motion sickness symptoms
Category | Symptom |
Dizzy | Dizziness, vertigo, dizziness, heavy head, head rotation, dizziness, instability, etc. |
Visual symptoms | Blurred vision, blurred objects in front of the eyes, reduced visual field, nystagmus, etc. |
Balance and gait | Unstable gait, difficulty walking, rocking gait, feeling unbalanced, easy falling, etc. |
Tinnitus | Tinnitus, buzzing, pressure in the ear, blocked ear, etc. |
Nausea and vomiting | Nausea, vomiting tendency, stomach upset, motion sickness nausea, etc. caused by rotational motion sickness |
Anxiety and fear | Worries about motion sickness symptoms, fear of going out or participating in activities, social phobia, etc. |
Other symptoms | Headache, muscle tension, fatigue, drowsiness, palpitations, insomnia, etc. |
Motion sickness is a significant concern in the field of vehicle engineering due to its substantial interindividual variability and potential impact on the comfort and safety of vehicle occupants. Sensitivity to motion sickness varies considerably across different age groups, with infants generally exhibiting lower sensitivity, while susceptibility begins in children aged 6-7 years and peaks at age 9 years [20]. the likelihood of experiencing motion sickness also varies significantly between genders, with women having a notably higher incidence compared to men [21]. It has been estimated that women are at least three times more likely than men to report being affected [22]. Speculations have arisen regarding the potential relationship between motion sickness occurrence and the female menstrual cycle [23]. Studies have indicated that hormone fluctuations may be associated with symptoms of motion sickness, suggesting that factors influencing this condition could potentially be linked to hormonal changes during a woman’s menstrual cycle [24]. Various factors influence motion sickness (such as age, gender, eating habits, and low-frequency vibration), some of which exhibit high individuality as depicted in Fig. 1.
Fig. 2Factors affecting motion sickness
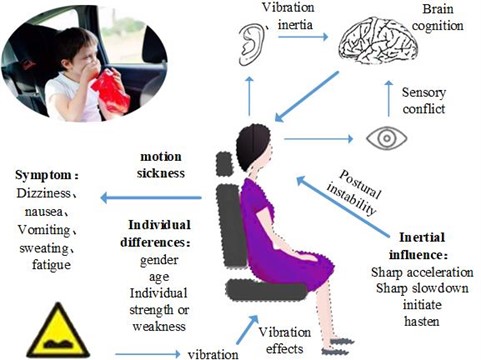
The close correlation between low-frequency vibration and motion sickness has been widely acknowledged by scholars. Low frequency vibration has a significant impact on the degree of motion sickness [25], especially low frequency vibration below 1 Hz [26], especially low frequency vibration below 0.5 Hz [27], which is more likely to cause motion sickness. Griffin et al. [28], pioneers in the field of motion sickness research derived a mathematical model for motion sickness through vibration experiments and summarized the relationship between vibration direction, frequency, amplitude, and motion sickness. Furthermore, Lawther introduced the frequency domain weighted representation method [29] to establish a link between the influence of motion sickness and sensitivity to low-frequency vibrations, thereby laying an important foundation for quantitative evaluation and prediction of motion sickness. These findings have been standardized in ISO 2631-1(1997) [30] and ISO 6841(1987) [31], which also consider the impact of human posture. Building upon this knowledge base, single-degree-of-freedom [32] and multi-degree-of-freedom [33] mathematical models related to the local activities of drivers and passengers have been further investigated.
2.2. Sickness in autonomous vehicles
Autonomous vehicles bring about changes in the driver’s role to passengers, especially driving behaviors such as rapid acceleration, rapid deceleration, and sharp turns, making passengers experience unaccustomed motion stimuli [34]. Large vibration acceleration not only reduces riding comfort but also easily causes motion sickness symptoms [35]. in terms of motion direction, the sensitivity of the human body to horizontal motion is slightly greater than that of vertical and pitching motion [36], that is, in complex motion with different motion directions but the same other elements (including multiple motion directions), the contribution rate of motion sickness is mainly due to horizontal vibration [37].
In the journey of autonomous vehicles, motion sickness is not only affected by vehicle acceleration and low-frequency vibration. As passengers have more options for free movement, this can also lead to visual stimulation and head tilt, producing VIMS and OKMS, which are also important factors in motion sickness [38]. The passengers of autonomous vehicles have more freedom of choice, which can exacerbate head movements, which increases the likelihood of motion sickness [39]. The main causes of dizziness are related to vestibular organ dysfunction or lack of coordination with other sensory systems [40]. The vestibular organs are a group of sensory organs located in the inner ear that detect the position, movement, and acceleration of the head. Vertigo may occur when the perception of motion received by the vestibular organs conflicts with the perception of motion received by other sensory systems, which is shown in Fig. 3.
Fig. 3Diagram of the vestibular system principles
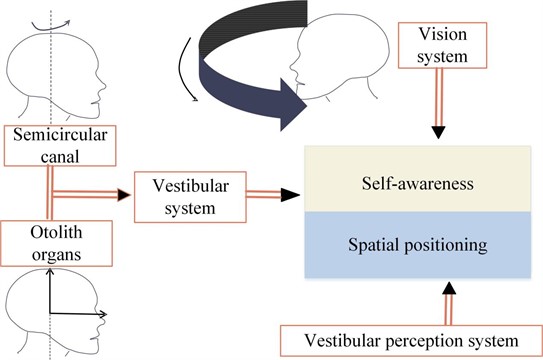
Passengers experience different types of motion sickness caused by visual motion scenes or simulated movements in a virtual environment. In autonomous vehicles, passengers have more time for their own activities, especially for entertainment or work. When passengers perform these activities during the journey, the head tilt causes Optical dynamic motion sickness (OKMS); and when the vision is stimulated, it produces Visually Induced Motion Sickness (VIMS) [41], which is considered a precursor to motion sickness. in autonomous vehicles, these two types of motion sickness are the main types in autonomous vehicles due to the increased autonomous activities of passengers. VIMS usually occurs when there is little or no physical movement. The eye tracker system is associated with continuous low-frequency head movements, which can maintain the image stability of the retina [42]. Nystagmus is the involuntary, rapid, repetitive movement of the eye, and the movement of large visual scenes induces visually-induced nystagmus, leading to convection and VIMS as shown in Fig. 3. in self-driving cars, VIMS is unlikely to occur because of the actual physical motion. However, VIMS should be considered when using virtual reality (VR) as an entertainment option for self-driving cars or to alleviate motion sickness. in order to mitigate motion sickness using an immersive approach, the movement of the virtual environment must be accurately synchronized with the actual movement of the vehicle, thus minimizing sensory conflict. Any inconsistency between sensory inputs increases the risk of motion sickness. a major challenge for autonomous vehicles is the limited availability of external visual stimuli, so eliminating sensory conflict in virtual and real environments is difficult. Optical dynamic motion sickness (OKMS) occurs when the head is rotated relative to the visual scene under the influence of the pseudo-Coriolis effect (PCE) [43]. The Coriolis effect (PCE) refers to a type of motion sickness that occurs when the head is tilted during body rotation [44]. The perceived rotation is caused by the rotation of the visual scene around a vertical axis, while the subject remains relatively still with respect to the scene [45]. Thus, perceived self-motion and head tilt can lead to PCE, resulting in motion sickness in virtual environments [46]. in self-driving cars, OKMS do not exist because passengers rarely participate in the spinning motion [47]. However, when passengers read or use electronic devices, they can encounter PCE due to a downward tilt of the head [48].
Fig. 4VIMS schematic diagram
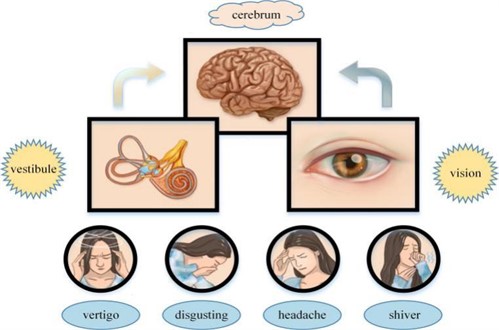
3. Detection of motion sickness
Evaluation methods play a pivotal role in motion sickness research, with medical professionals typically employing a combination of clinical assessment and observation to obtain a comprehensive understanding of motion sickness symptoms. Although there are no specific medical standards for motion sickness, various tools and methodologies can be utilized to assess and comprehend its symptoms. Table 2 provides an overview of the detection methods and classification of dizziness measurement standards.
Table 2Dizziness detection methods table
Evaluation methodology | Description |
Questionnaire tool [49] | 1. SSQ questionnaire 2. MSQ questionnaire |
Rapid motion sickness (FMS) scale [52] | It is used to quickly measure the extent of motion sickness symptoms during exposure, with subjects verbally assessing their feelings on a minute-by-minute basis |
Physiological signals [56] | Heart rate variability (HRV), Electro gastric photogram (EGG), Skin conductance, electrocardiogram (ECG) electroencephalogram (EEG), Vestibulo-Ocular Reflex (VOR) |
Posture stability [62] | People prone to motion sickness have more unstable posture without considering visual and sensory feedback |
3.1. Subjective evaluation method
Subjective evaluation methods mainly include questionnaire survey and symptom diagnosis.
In 1975, Golding proposed a Motion Sickness Susceptibility Questionnaire (MSSQ). This questionnaire assesses an individual's susceptibility to motion sickness based on whether the individual has experienced motion sickness in real life and the frequency of its occurrence [49]. in 2006, the MSQ was simplified, and the accuracy of the simplified MMSQ was proved to be no less than that of the original, and the simplified MSSQ is now mainly used [50]. MSQ is easy to operate, economical easy to implement, and has a certain accuracy. in response to motion sickness caused by VIMS, Kennedy modified the MSSQ survey form and established the SSQ survey form to enhance the ability to identify “problem” simulators and improve diagnostic capabilities [51].
However, the two questionnaire methods for appeals are based on empirical approaches following the onset of motion sickness, lacking a real-time evaluation method. To address this gap, Keshavarz et al introduced the Rapid Motion Sickness Scale (FMS) [52] to enable real-time measurement and rapid assessment of motion sickness [53]. Participants were asked to verbally rate the severity of their motion sickness on a 20-point scale at regular intervals. Nevertheless, FMS does not play a pivotal role in assessing motion sickness and has certain limitations [54].
The diagnosis of symptoms primarily relies on the observation of clinical manifestations and signs in patients to determine the severity of motion sickness. The evaluation criteria mainly encompass vomiting, vertigo, and postural stability [55]. Currently, a prevalent method for symptom diagnosis involves comprehensive assessment based on diverse symptoms and signs associated with human motion sickness.
The subjective evaluation method possesses the advantages of convenience, cost-effectiveness, and widespread adoption, making it a continuously evolving approach. However, its ability to accurately predict the nonlinear changes in motion sickness severity over time remains limited.
3.2. Physiological signals
The objective measurement method aims to assess the severity of motion sickness by quantifying individual physiological indicators.
Electrogastrogram (EGG) is utilized for detecting alterations in stomach activity during the onset of motion sickness [56]. EGG changes are highly sensitive when motion sickness occurs (EEG activity increases significantly), thus it can be employed to assess the severity of MS symptoms [57]. However, EGG alone cannot serve as an indicator of MS severity due to its susceptibility to various factors. More accurate outcomes can be achieved by integrating other measurement techniques.
Heart rate variability (HRV) refers to the extent of fluctuation in intervals between consecutive heartbeats, which is assessed by analyzing the temporal gap between adjacent heartbeats. This physiological parameter serves as an indicator of the autonomic nervous system's functional equilibrium. Motion sickness induced by vestibule-visual conflict has been associated with an elevation in HRV [58], wherein increased sympathetic nerve activity and decreased vagus nerve activity are observed with escalating severity of motion sickness [59].
Electroencephalogram (EEG) is the electrical signal generated by the activity of neurons in the brain. with the occurrence of motion sickness, the central motor area, sensory area, and visual area showed a trend of increasing power spectral density centroid frequency in EEG signals [60], while and power in the motor area, parietal lobe and occipital lobe also increased significantly, and the increase was positively correlated with the degree of individual discomfort [61]. Therefore, EEG provides an objective reference for evaluating the severity of motion sickness.
Postural stability assessment is often used to measure an individual's ability to control and balance their body in different postures, which is crucial to understanding the mechanism of motion sickness. When visual and sensory feedback were excluded, postural stability predicted the severity of motion sickness. After the journey, the more severe the motion sickness, the worse the postural stability [62]. Patients with visually induced motion sickness, due to excessive visual input, are more prone to symptoms of motion sickness [63], which may include vertigo, dizziness, nausea, and other symptoms, because excessive visual information may conflict with the input of other sensory systems, aggravating the performance of motion sickness [64]. It is worth noting that there are significant individual differences in the assessment of postural stability.
The Vestibulo-Ocular Reflex (VOR) is a physiological mechanism that regulates eye movement to maintain visual stability by perceiving and compensating for head movements [65]. Motion sickness often arises from dysfunction in the vestibular system, making VOR testing crucial for assessing vestibular function and evaluating the adequacy of eye responses during head movements [66]. in patients presenting with motion sickness symptoms, alterations in the vestibular eye movement reflex may manifest as follows: 1. Abnormal eye tracking: Eye tracking may become erratic or inaccurate when the patient moves their head, resulting in unstable visual images due to ineffective eyeball following [67]. 2. Delayed or inadequate response: Normally, VOR promptly adjusts eye position to counteract visual instability caused by head movement [65]; however, during motion sickness onset, this response may be delayed or insufficient [68].
Objective evaluation methods typically involve the comprehensive measurement of human physiological indicators; however, relying solely on these indicators may not accurately depict motion sickness due to individual differences that influence changes in physiological signals, leading to a lack of statistical significance in measurement results. Therefore, integrating these metrics with other approaches is a prudent choice. As of now, there is no established “gold standard” for diagnostic test methods and measurement standards for motion sickness. Hence, it is imperative to employ diverse methodologies and consider multiple outcomes in the comprehensive assessment of motion sickness.
4. Relieves motion sickness
Currently, we can explore avenues to alleviate motion sickness induced by autonomous driving from both passenger and vehicle perspectives. The distinctive attributes of autonomous vehicles render them more prone to inducing motion sickness. Fig. 6 presents an analysis of the impact of autonomous vehicles on motion sickness based on their inherent characteristics.
Fig. 5The relationship between autonomous vehicles and motion sickness
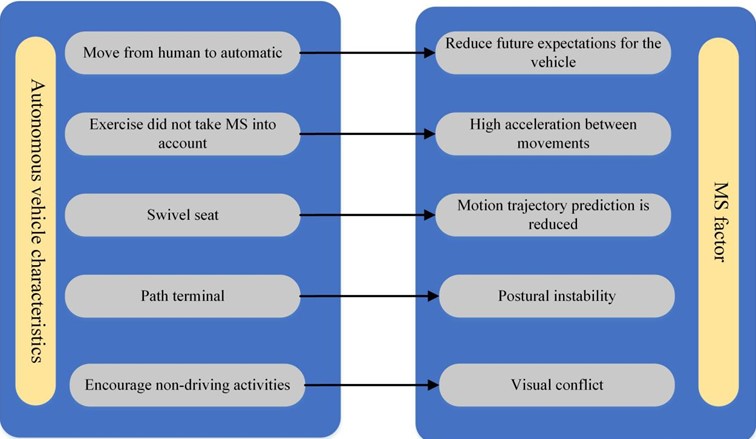
4.1. Passenger-centered relief motion sickness
By providing passengers with advanced vehicle and road information, it aids in mitigating the occurrence of motion sickness. This approach aligns with the sensory conflict hypothesis and encompasses the acquisition of visual, auditory, and tactile cues.
Tal D, Gonen A, and Wiener G observed a significant increase in motion sickness when the visual field was blocked. to effectively reduce the incidence of motion sickness [69], researchers suggest providing passengers with an unobstructed visual field environment. Turner M. and Griffin M. J. conducted numerous long-distance travel experiments and concluded that enhancing passengers’ forward visual field can greatly decrease the occurrence of motion sickness [71]. C. R. Gordon and O. Spitzer’s research on seasickness also reached a similar conclusion [70]. Therefore, Diels and Bos proposed placing displays near passenger windows to allow them to perceive their surroundings, reducing sensory conflict while obtaining entertainment information [72]. Bos and Kinnon propose displaying real-time road views to passengers through screens [73], enabling access to road information for passengers. Tal D. suggests informing passengers about their surroundings as a means to reduce sensory conflict [74]. When using displays to provide a comfortable visual field and monitor movement information, it is important to select appropriate size and positioning options [75]. Utilizing virtual reality devices to create synchronized sight between passengers’ vision and vehicle movement scenes through visual input [76], ensuring consistency between their visual cues, vestibular system, and sensory input can alleviate motion sickness symptoms [77]; this method holds promise. Shielding visual input can also significantly alleviate motion sickness; however, it is not a complete solution [78].
Traditional car drivers typically do not experience motion sickness due to their ability to anticipate the vehicle's driving path and state in advance, actively adjusting their heads and upper bodies towards the direction of rotation [79]. However, when transitioning from a driver to a passenger role in autonomous driving [80], this predictive capability diminishes, thereby increasing the risk of motion sickness [81]. Consequently, enhancing passengers’ trajectory prediction abilities and reducing the likelihood of motion sickness in autonomous driving can be achieved by providing advanced indications about future vehicle movement directions through internal audio systems for guiding responses [82], or utilizing wearable devices employing various vibration methods to alert passengers about upcoming operations [83], [84]. Bjka and Bnmya suggest that expanding window areas and using elevated seats can offer a broader and clearer field of vision, ensuring clear visibility of roads with visual cues for vehicle trajectories, thus minimizing the occurrence of motion sickness [85].
4.2. Vehicle-centered motion sickness relief
This paper analyzes the influence of vehicle factors on motion sickness, as shown in Fig. 7.
Fig. 6The influence of vehicle factors on motion sickness
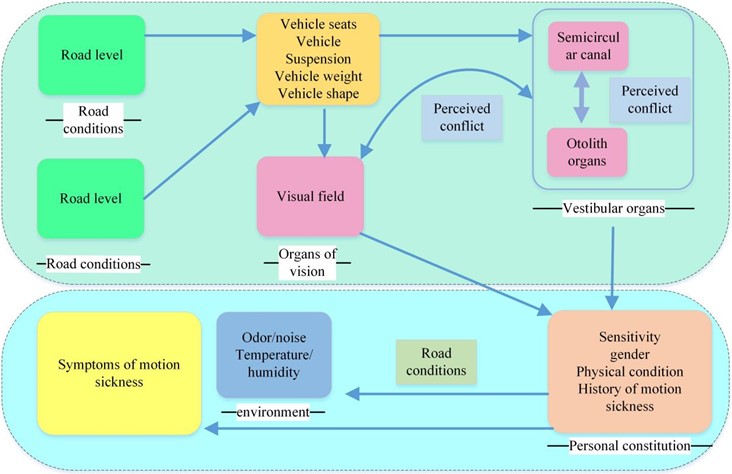
The occurrence of motion sickness in autonomous vehicles can be reduced by optimizing the motion planning algorithm and selecting routes with good traffic conditions [86], as speed, vertical vibration, lateral acceleration, and horizontal acceleration have been found to impact motion sickness. Waymo’s research team suggests that avoiding traffic congestion can minimize rapid vehicle accelerations and decelerations, thereby reducing the incidence of motion sickness [88]. Simulating the smooth driving style of an experienced driver can also help alleviate motion sickness [89]. Additionally, many scholars have utilized suspension systems to mitigate vehicle vibrations and subsequently reduce motion sickness [90]. by employing control optimization techniques such as fuzzy PID control algorithm [91] or LQG control [92], low-frequency vibrations within the range of 0.1-0.5 Hz can be avoided while improving ride comfort and minimizing car-induced motion sickness occurrences [93]. Furthermore, active suspension control optimization has shown the potential to reduce the likelihood of experiencing motion sickness by achieving a more stable driving condition through controlling vertical acceleration [94], pitch acceleration [95], and roll Angle acceleration [96].
In terms of vehicle materials, piezoelectric metamaterials show a unique ability to effectively control the amplitude and direction of wave propagation. Taking this property into account, the use of piezoelectric metamaterials to improve the material of vehicle chassis has become an interesting option. By applying piezoelectric metamaterials to the chassis, the phenomenon of low-frequency vibration transmission to the passenger area can be effectively reduced. This method is not only expected to improve the comfort of passengers but also to improve the overall performance and driving experience of the vehicle [97]. The special properties of piezoelectric metamaterials make them have broad application prospects in vehicle engineering and provide new possibilities for reducing vibration transmission and improving ride quality.
By optimizing the structural design of vehicle chassis, the transmission of low-frequency vibration from road excitation to passengers through suspension can be effectively mitigated, thereby reducing the incidence of motion sickness [98].
By integrating sensors that record passengers’ physiological signals and combining them with vehicle movement data, Jaguar Land Rover researchers have provided personalized autonomous driving capabilities. Give each passenger a health score and establish a basic set of driving parameters based on matching Settings, eliminating the problem of different passengers having to adjust different data. When passengers are working or relaxing in the car, this method can reduce the incidence of motion sickness by 60 % [99]. Adapting self-driving cars to passenger needs to achieve personalization and adapt to different driver and passenger seat positions can also reduce motion sickness. The occurrence of motion sickness is also affected by the direction of the seat [100]. Changing the direction of the seat to make it parallel to the direction of the front and back movement of the body and the direction of stomach peristalsis can effectively prevent the occurrence of motion sickness [101].
5. Conclusions
On the basis of an in-depth discussion of relevant literature in recent years, this article comprehensively summarizes the current theoretical methods and technologies for alleviating motion sickness, and proposes the following three conclusions and future prospects:
1) The existing research on the pathogenesis of motion sickness is mainly based on sensory conflicts and mostly adopts medical models. However, there is a lack of appropriate vehicle-related models to quantify the degree of motion sickness among passengers. Therefore, it is necessary to explore the mechanism and quantitative relationship between the motion state of autonomous vehicles and motion sickness, establish the corresponding motion sickness mechanism model of autonomous vehicles, and provide a theoretical basis for further research on motion sickness caused by autonomous vehicles.
2) In the research field of motion sickness in autonomous vehicles, subjective evaluation is still the main method of motion sickness detection. This method has the advantages of simple implementation, low cost, and high effectiveness, but it suffers from strong randomness and poor real-time performance. Although objective measurement methods have real-time characteristics, they are complex to operate, costly, and have poor stability. Therefore, the best choice for measurement methods is a combination of subjectivity and objectivity. in addition, the current lack of unified standards to measure the severity of motion sickness is also a problem. Establishing a unified evaluation standard for motion sickness in autonomous driving will greatly promote the exploration of motion sickness discomfort in autonomous driving technology.
3) At present, the methods to alleviate motion sickness in autonomous driving mainly focus on expanding the passenger's field of view and optimizing the interior environment and seats. These methods are effective in reducing motion sickness symptoms in both autonomous driving and traditional vehicles. However, the research on the optimization of driving routes and attitude control algorithms using the controllable characteristics of an autonomous vehicle's motion state is relatively limited and has not been effectively verified in practice. Therefore, improving passenger comfort and reducing stimulation may be a promising direction for alleviating motion sickness in autonomous driving.
However, there are certain limitations to this study. Firstly, it predominantly relies on a comprehensive literature review and the synthesis of relevant studies, without incorporating empirical research. Secondly, numerous unanswered questions and areas necessitating further investigation in the realm of autonomous vehicles persist, such as the standardization of motion sickness mechanisms and evaluation methodologies. Consequently, future studies should delve deeper into these issues and employ empirical research methods for validation purposes. in summary, enhancing the development and mitigation of motion sickness in autonomous vehicles will not only enhance passenger comfort but also bolster public acceptance of autonomous vehicles while yielding substantial societal and economic benefits.
References
-
M. Clavijo, F. Jiménez, and J. E. Naranjo, “The development and prospects of autonomous driving technology,” Applied Sciences, Vol. 13, No. 9, p. 5377, Apr. 2023, https://doi.org/10.3390/app13095377
-
A. Brietzke, T. Kantusch, R. Pham Xuan, A. Dettmann, S. Marker, and A. C. Bullinger, “What can user typologies tell us about carsickness criticality in future mobility systems,” SAE International Journal of Connected and Automated Vehicles, Vol. 5, No. 2, pp. 135–145, Feb. 2022, https://doi.org/10.4271/12-05-02-0012
-
L. Brooke, “SAE and ISO refine the levels of driving automation,” Automotive Engineering, Vol. 5, No. 8, 2021.
-
“2023-2029 Global and China kinetosis drugs industry research and 14sdth five year plan analysis report,” China, 2023.
-
J. Yeong, G. Velasco-Hernandez, J. Barry, and J. Walsh, “Sensor and sensor fusion technology in autonomous vehicles: a review,” Sensors, Vol. 21, No. 6, p. 2140, Mar. 2021, https://doi.org/10.3390/s21062140
-
J. Lee, D. Shiotsuka, T. Nishimori, K. Nakao, and S. Kamijo, “GAN-Based LiDAR Translation between sunny and adverse weather for autonomous driving and driving simulation,” Sensors, Vol. 22, No. 14, p. 5287, Jul. 2022, https://doi.org/10.3390/s22145287
-
K. Jo, Y. Jo, J. K. Suhr, H. G. Jung, and M. Sunwoo, “Precise localization of an autonomous car based on probabilistic noise models of road surface marker features using multiple cameras,” IEEE Transactions on Intelligent Transportation Systems, Vol. 16, No. 6, pp. 3377–3392, Dec. 2015, https://doi.org/10.1109/tits.2015.2450738
-
S. Atifah Saruchi, N. A. Izni, M. H. M. Ariff, and N. Wahid, “A brief review on motion sickness for autonomous vehicle,” Enabling Industry 4.0 through Advances in Mechatronics, pp. 275–284, May 2022, https://doi.org/10.1007/978-981-19-2095-0_24
-
K. Young, M. Regan, and M. Hammer, “Driver distraction: a review of the literature,” Monash University Accident Research Centre, Report #206, 2003.
-
M. Paula, “Self-driving cars can be relaxing, as the rinspeed xchange concept shows,” Applied Ergonomics, Vol. 53, pp. 374–382, 2016.
-
V. G. Macefield, “Developments in autonomic research: a review of the latest literature,” Clinical Autonomic Research, Vol. 19, No. 1, pp. 3–5, Feb. 2009, https://doi.org/10.1007/s10286-009-0520-5
-
S. Teng et al., “Motion planning for autonomous driving: the state of the art and future perspectives,” IEEE Transactions on Intelligent Vehicles, Vol. 8, No. 6, pp. 3692–3711, Jun. 2023, https://doi.org/10.1109/tiv.2023.3274536
-
J. F. Golding and M. A. Gresty, “Motion sickness,” Current Opinion in Neurology, Vol. 18, pp. 29–34, 2005.
-
A. E. Arnold et al., “Human spatial orientation, neural basis of,” International Encyclopedia of the Social and Behavioral Sciences, Vol. 238, pp. 386–391, Jan. 2015, https://doi.org/10.1016/b978-0-08-097086-8.56012-7
-
B. E. Donohew, “Motion sickness with lateral and roll oscillation,” Ph.D. Thesis, University of Southampton, Institute of Sound and Vibration Research, 2006.
-
L. Jiang, “A preliminary study on the human body movement perception flight environment,” Nanjing University of Aeronautics and Astronautics, 2024.
-
J. T. Reason, “Motion sickness adaptation: a neural mismatch model,” Journal of the Royal Society of Medicine, Vol. 71, No. 11, pp. 819–829, Mar. 2018, https://doi.org/10.1177/014107687807101109
-
M. Treisman, “Motion sickness: an evolutionary hypothesis,” Science, Vol. 197, No. 4302, pp. 493–495, Jul. 1977, https://doi.org/10.1126/science.301659
-
T. A. Stoffregen and L. J. Smart, “Postural instability precedes motion sickness,” Brain Research Bulletin, Vol. 47, No. 5, pp. 437–448, Nov. 1998, https://doi.org/10.1016/s0361-9230(98)00102-6
-
I. F. Henriques, D. W. Douglas de Oliveira, F. Oliveira-Ferreira, and P. M. O. Andrade, “Motion sickness prevalence in school children,” European Journal of Pediatrics, Vol. 173, No. 11, pp. 1473–1482, Jun. 2014, https://doi.org/10.1007/s00431-014-2351-1
-
Moira B. Flanagan, James G. May, and Thomas G. Dobie, “Sex differences in tolerance to visually-induced motion sickness,” Aviation, Space, and Environmental Medicine, Vol. 76, No. 7, pp. 642–646, Jul. 2005.
-
F. Koslucher, E. Haaland, and T. A. Stoffregen, “Sex differences in visual performance and postural sway precede sex differences in visually induced motion sickness,” Experimental Brain Research, Vol. 234, No. 1, pp. 313–322, Oct. 2015, https://doi.org/10.1007/s00221-015-4462-y
-
R. L. Matchock, M. E. Levine, P. J. Gianaros, and R. M. Stern, “Susceptibility to nausea and motion sickness as a function of the menstrual cycle,” Women’s Health Issues, Vol. 18, No. 4, pp. 328–335, Jul. 2008, https://doi.org/10.1016/j.whi.2008.01.006
-
John F. Golding, Priscilla Kadzere, and Michael A. Gresty, “Motion sickness susceptibility fluctuates through the menstrual cycle,” Aviation, space, and environmental medicine, Vol. 76, No. 10, pp. 970–973, Oct. 2005.
-
B. E. Donohew and M. J. Griffin, “Report on analysis of SNCF train field tests regarding motion sickness,” Deliverable, 2005.
-
J. T. Reason and J. J. Brand, “Motion Sickness.,” the American Journal of Psychology, Vol. 90, No. 1, pp. 33–82, 1977.
-
H. Suzuki, H. Shiroto, and K. Tezuka, “Effects of low frequency vibration on train motion sickness,” Quarterly Report of RTRI, Vol. 46, No. 1, pp. 35–39, Jan. 2005, https://doi.org/10.2219/rtriqr.46.35
-
B. Donohew and M. Griffin, “Effect of the frequency of combined lateral and roll oscillation on motion sickness: report on laboratory experiment 1 conducted by ISVR.deliverable,” 2004.
-
J. F. O’Hanlon, and M. E. Mccauley, “Motion sickness incidence as a function of the frequency and acceleration of vertical sinusoidal motion,” Aerospace Medicine, Vol. 45, No. 4, pp. 366–369, Apr. 1974.
-
“Mechanical vibration and shock-Evaluation of human exposure to whole-body vibration-Part 4: Guidelines for the evaluation of the effects of vibration and rotational motion on passenger and crew comfort in fixed-guideway transport systems,” ISO 2631-1, 1997.
-
“Evaluation of Human Exposure to Whole-body Vibration. Part 1,” ISO-2631/1, 1997.
-
“Measurement and evaluation of human exposure to whole-body mechanical vibration and shock,” BS 6841:1987, 1987.
-
M. J. Griffin and K. L. Mills, “Effect of magnitude and direction of horizontal oscillation on motion sickness,” Aviation Space and Environmental Medicine, Vol. 73, No. 7, pp. 640–646, 2002.
-
J. F. Golding, “Motion sickness,” Handbook of Clinical Neurology, Vol. 137, pp. 371–390, Jan. 2016, https://doi.org/10.1016/b978-0-444-63437-5.00027-3
-
T. Irmak, V. Kotian, R. Happee, K. N. de Winkel, and D. M. Pool, “Amplitude and temporal dynamics of motion sickness,” Frontiers in Systems Neuroscience, Vol. 16, p. 866503, May 2022, https://doi.org/10.3389/fnsys.2022.866503
-
J. F. Golding and H. M. Markey, “Effect of frequency of horizontal linear oscillation on motion sickness and somatogravic illusion,” Aviation, Space, and Environmental Medicine, Vol. 67, No. 2, pp. 121–126, Feb. 1996.
-
M. J. Griffin and M. M. Newman, “An experimental study of low-frequency motion in cars,” Proceedings of the Institution of Mechanical Engineers, Part D: Journal of Automobile Engineering, Vol. 218, No. 11, pp. 1231–1238, Nov. 2004, https://doi.org/10.1243/0954407042580093
-
C. Butler and M. J. Griffin, “Motion sickness with combined fore-aft and pitch oscillation: effect of phase and the visual scene,” Aviation, Space, and Environmental Medicine, Vol. 80, No. 11, pp. 946–954, Nov. 2009, https://doi.org/10.3357/asem.2490.2009
-
K. E. Money, “Motion sickness,” Physiological Reviews, Vol. 50, No. 1, pp. 1–39, Jan. 1970, https://doi.org/10.1152/physrev.1970.50.1.1
-
M. I. Boldingh, U. Ljøstad, Mygland, and P. Monstad, “Vestibular sensitivity in vestibular migraine: VEMPs and motion sickness susceptibility,” Cephalalgia, Vol. 31, No. 11, pp. 1211–1219, Jul. 2011, https://doi.org/10.1177/0333102411409074
-
D. B. Lawson, “Technology management and user acceptance of virtual environment technology,” in Handbook of Virtual Environments, CRC Press, 2014, pp. 523–534, https://doi.org/10.1201/b17360-29
-
R. J. Leigh and D. S. Zee, “The neurology of eye movements,” Optometry and Vision Science, Vol. 61, No. 2, pp. 139–140, Jun. 2015, https://doi.org/10.1093/med/9780199969289.001.0001
-
J. Dichgans and T. Brandt, “Optokinetic motion sickness and pseudo-Coriolis effects induced by moving visual stimuli,” Acta Oto-Laryngologica, Vol. 76, No. 1-6, pp. 339–348, Jul. 2009, https://doi.org/10.3109/00016487309121519
-
J. R. Lackner and P. Dizio, “Gravitational, inertial, and Coriolis force influences on nystagmus, motion sickness, and perceived head trajectory,” in the Head-Neck Sensory Motor System, Oxford University PressNew York, 1992, pp. 216–222, https://doi.org/10.1093/acprof:oso/9780195068207.003.0033
-
K. M. T. Pöhlmann, J. Föcker, P. Dickinson, A. Parke, and L. O. ’Hare, “The relationship between vection, cybersickness and head movements elicited by illusory motion in virtual reality,” Displays, Vol. 71, p. 102111, Jan. 2022, https://doi.org/10.1016/j.displa.2021.102111
-
J. Iskander, M. Hossny, and S. Nahavandi, “A review on ocular biomechanic models for assessing visual fatigue in virtual reality,” IEEE Access, Vol. 6, pp. 19345–19361, Jan. 2018, https://doi.org/10.1109/access.2018.2815663
-
R. Ford, “Vehicle Tracking: State of the art,” Transportion Research Board, Vol. 55, No. 592, pp. 25–27, 1998.
-
W. Bles, “Coriolis effects and motion sickness modelling,” Brain Research Bulletin, Vol. 47, No. 5, pp. 543–549, Nov. 1998, https://doi.org/10.1016/s0361-9230(98)00089-6
-
J. F. Golding, “Predicting individual differences in motion sickness susceptibility by questionnaire,” Personality and Individual Differences, Vol. 41, No. 2, pp. 237–248, Jul. 2006, https://doi.org/10.1016/j.paid.2006.01.012
-
B. Keshavarz, B. Murovec, N. Mohanathas, and J. F. Golding, “The visually induced motion sickness susceptibility questionnaire (VIMSSQ): estimating individual susceptibility to motion sickness-like symptoms when using visual devices,” Human Factors: the Journal of the Human Factors and Ergonomics Society, Vol. 65, No. 1, pp. 107–124, Apr. 2021, https://doi.org/10.1177/00187208211008687
-
R. S. Kennedy, N. E. Lane, K. S. Berbaum, and M. G. Lilienthal, “Simulator sickness questionnaire: an enhanced method for quantifying simulator sickness,” the International Journal of Aviation Psychology, Vol. 3, No. 3, pp. 203–220, Jul. 1993, https://doi.org/10.1207/s15327108ijap0303_3
-
K. M. Stanney, K. S. Hale, I. Nahmens, and R. S. Kennedy, “What to expect from immersive virtual environment exposure: influences of gender, body mass index, and past experience,” Human Factors: the Journal of the Human Factors and Ergonomics Society, Vol. 45, No. 3, pp. 504–520, Aug. 2016, https://doi.org/10.1518/hfes.45.3.504.27254
-
“Taxonomy and definitions for terms related to on-road motor vehicle automated driving systems,” SAE Standard J3016, 2014.
-
B. Keshavarz and H. Hecht, “Validating an efficient method to quantify motion sickness,” Human Factors: the Journal of the Human Factors and Ergonomics Society, Vol. 53, No. 4, pp. 415–426, Apr. 2011, https://doi.org/10.1177/0018720811403736
-
V. Sevinc and M. I. Berkman, “Psychometric evaluation of simulator sickness questionnaire and its variants as a measure of cybersickness in consumer virtual environments,” Applied Ergonomics, Vol. 82, p. 102958, Jan. 2020, https://doi.org/10.1016/j.apergo.2019.102958
-
Z. Nachum, C. R. Gordon, B. Shahal, O. Spitzer, and A. Shupak, “Active high‐frequency vestibulo‐ocular reflex and seasickness susceptibility,” the Laryngoscope, Vol. 112, No. 1, pp. 179–182, Jan. 2009, https://doi.org/10.1097/00005537-200201000-00031
-
S. Q. Hu, K. A. Mcchesney, and K. A. Player, “Systematic investigation of physiological correlates of motion sickness induced by viewing an optokinetic rotating drum,” Aviation Space and Environmental Medicine, Vol. 70, No. 8, pp. 759–765, 1999.
-
A. H. X. Yang, P. Khwaounjoo, and Y. O. Cakmak, “Directional effects of whole-body spinning and visual flow in virtual reality on vagal neuromodulation,” Journal of Vestibular Research, Vol. 31, No. 6, pp. 479–494, Oct. 2021, https://doi.org/10.3233/ves-201574
-
C.-L. Lin, T.-P. Jung, S.-W. Chuang, J.-R. Duann, C.-T. Lin, and T.-W. Chiu, “Self-adjustments may account for the contradictory correlations between HRV and motion-sickness severity,” International Journal of Psychophysiology, Vol. 87, No. 1, pp. 70–80, Jan. 2013, https://doi.org/10.1016/j.ijpsycho.2012.11.003
-
Avi Shupak and Carlos R. Gordon, “Motion sickness: advances in pathogenesis, prediction, prevention, and treatment,” Aviation, Space, and Environmental Medicine, Vol. 77, No. 12, pp. 1213–1223, Dec. 2006.
-
L. A. Arwick-Evans, R. E. Church, and C. Hancock, “Electrodermal activity as an index of motion sickness,” Aviation Space and Environmental Medicine, Vol. 58, No. 5, 1987.
-
L. J. Smart, T. A. Stoffregen, and B. G. Bardy, “Visually induced motion sickness predicted by postural instability,” Human Factors: the Journal of the Human Factors and Ergonomics Society, Vol. 44, No. 3, pp. 451–465, Sep. 2016, https://doi.org/10.1518/0018720024497745
-
N. Owen, A. G. Leadbetter, and L. Yardley, “Relationship between postural control and motion sickness in healthy subjects,” Brain Research Bulletin, Vol. 47, No. 5, pp. 471–474, Nov. 1998, https://doi.org/10.1016/s0361-9230(98)00101-4
-
Y. Yokota, M. Aoki, K. Mizuta, Y. Ito, and N. Isu, “Motion sickness susceptibility associated with visually induced postural instability and cardiac autonomic responses in healthy subjects,” Acta Oto-Laryngologica, Vol. 125, No. 3, pp. 280–285, Jul. 2009, https://doi.org/10.1080/00016480510003192
-
H. Sato, Y. Sato, and T. Wada, “Relationship between motion sickness and accuracy of vestibulo-ocular reflex,” in Proceedings of the Human Factors and Ergonomics Society Annual Meeting, Vol. 64, No. 1, pp. 765–769, Feb. 2021, https://doi.org/10.1177/1071181320641176
-
M. Cherchi, “Utricular function in vestibular neuritis: a pilot study of concordance/discordance between ocular vestibular evoked myogenic potentials and ocular cycloposition,” Experimental Brain Research, Vol. 237, No. 6, pp. 1531–1538, Mar. 2019, https://doi.org/10.1007/s00221-019-05529-8
-
G. Bertolini and D. Straumann, “Moving in a moving world: a review on vestibular motion sickness,” Frontiers in Neurology, Vol. 7, p. 175198, Feb. 2016, https://doi.org/10.3389/fneur.2016.00014
-
G. Bertolini, A. Pagnamenta, A. Kunz, A. Del Torso, and D. Bron, “Reduction of the vertical vestibular-ocular reflex in military aircraft pilots exposed to tactical, high-performance flight,” Frontiers in Neurology, Vol. 14, p. 949227, Jun. 2023, https://doi.org/10.3389/fneur.2023.949227
-
D. Tal et al., “Artificial horizon effects on motion sickness and performance,” Otology and Neurotology, Vol. 33, No. 5, pp. 878–885, Jul. 2012, https://doi.org/10.1097/mao.0b013e318255ddab
-
R. Gordon, O. Spitzer, and I. Doweck, “The vestibulo-ocular reflex and seasickness susceptibility,” Journal of Vestibular Research, Vol. 6, No. 4, pp. 229–233, 1996.
-
M. Turner and M. J. Griffin, “Motion sickness in public road transport: the relative importance of motion, vision and individual differences,” British Journal of Psychology, Vol. 90, No. 4, pp. 519–530, Dec. 2010, https://doi.org/10.1348/000712699161594
-
C. Diels and J. E. Bos, “User interface considerations to prevent self-driving carsickness,” in AutomotiveUI ’15: the 7th International Conference on Automotive User Interfaces and Interactive Vehicular Applications, pp. 14–19, Sep. 2015, https://doi.org/10.1145/2809730.2809754
-
J. Bos, S. Kinnon, and A. Patterson, “Motion sickness symptoms in a ship motion simulator: effects of inside, outside, and no view,” Aviation Space and Environmental Medicine, Vol. 76, No. 12, pp. 1111–1118, 2005.
-
D. Tal, G. Wiener, and A. Shupak, “Mal de debarquement, motion sickness and the effect of an artificial horizon,” Journal of Vestibular Research, Vol. 24, No. 1, pp. 17–23, Feb. 2014, https://doi.org/10.3233/ves-130505
-
C. Liu, D. Thompson, M. J. Griffin, and M. Entezami, “Effect of train speed and track geometry on the ride comfort in high-speed railways based on ISO 2631-1,” Proceedings of the Institution of Mechanical Engineers, Part F: Journal of Rail and Rapid Transit, Vol. 234, No. 7, pp. 765–778, Aug. 2019, https://doi.org/10.1177/0954409719868050
-
M. Mcgill, A. Ng, and S. A. Brewster, “How visual motion cues can influence sickness for in-car VR,” in CHI ’17: CHI Conference on Human Factors in Computing Systems, p. 469, May 2017, https://doi.org/10.1145/3027063.3049790
-
C. Diels, J. E. Bos, K. Hottelart, and P. Reilhac, “Motion sickness in automated vehicles: the elephant in the room,” Road Vehicle Automation 3, pp. 121–129, Jul. 2016, https://doi.org/10.1007/978-3-319-40503-2_10
-
S. Ishak, A. Bubka, and F. Bonato, “Visual occlusion decreases motion sickness in a flight simulator,” Perception, Vol. 47, No. 5, pp. 521–530, Feb. 2018, https://doi.org/10.1177/0301006618761336
-
A. J. C. Reuten, J. B. J. Smeets, J. Rausch, M. H. Martens, E. A. Schmidt, and J. E. Bos, “The (in)effectiveness of anticipatory vibrotactile cues in mitigating motion sickness,” Experimental Brain Research, Vol. 241, No. 5, pp. 1251–1261, Mar. 2023, https://doi.org/10.1007/s00221-023-06596-8
-
X. Wang, X. Ye, D. Hurwitz, X. Wang, C. Schost, and D. Noyce, “Effect of motion cues on highway driving performance in simulated driving,” Transportation Research Record: Journal of the Transportation Research Board, Vol. 2677, No. 6, pp. 93–112, Jan. 2023, https://doi.org/10.1177/03611981221144300
-
I. Doweck et al., “Alterations in R-R variability associated with experimental motion sickness,” Journal of the Autonomic Nervous System, Vol. 67, No. 1-2, pp. 31–37, Dec. 1997, https://doi.org/10.1016/s0165-1838(97)00090-8
-
O. Carsten and M. H. Martens, “How can humans understand their automated cars? HMI principles, problems and solutions,” Cognition, Technology and Work, Vol. 21, No. 1, pp. 3–20, May 2018, https://doi.org/10.1007/s10111-018-0484-0
-
N. Md. Yusof, J. Karjanto, J. M. B. Terken, F. L. M. Delbressine, and G. W. M. Rauterberg, “Gaining situation awareness through a vibrotactile display to mitigate motion sickness in fully-automated driving cars,” International Journal of Automotive and Mechanical Engineering, Vol. 17, No. 1, pp. 7771–7783, Apr. 2020, https://doi.org/10.15282/ijame.17.1.2020.23.0578
-
O. X. Kuiper, J. E. Bos, C. Diels, and E. A. Schmidt, “Knowing what’s coming: anticipatory audio cues can mitigate motion sickness,” Applied Ergonomics, Vol. 85, No. 6, p. 103068, May 2020, https://doi.org/10.1016/j.apergo.2020.103068
-
J. Karjanto, N. Md. Yusof, C. Wang, J. Terken, F. Delbressine, and M. Rauterberg, “The effect of peripheral visual feedforward system in enhancing situation awareness and mitigating motion sickness in fully automated driving,” Transportation Research Part F: Traffic Psychology and Behaviour, Vol. 58, pp. 678–692, Oct. 2018, https://doi.org/10.1016/j.trf.2018.06.046
-
Z. Liangliang, L. I. Dong, and J. Yifei, “Route and speed optimization fallback mechanism for automatic drive vehicle,” JP2019128962A, 2018.
-
E. H. Henry, C. Bougard, C. Bourdin, and L. Bringoux, “Car sickness in real driving conditions: Effect of lateral acceleration and predictability reflected by physiological changes,” Transportation Research Part F: Traffic Psychology and Behaviour, Vol. 97, pp. 123–139, Aug. 2023, https://doi.org/10.1016/j.trf.2023.06.018
-
D. L. Larner and J. S. Russell, “Method and system for deter⁃mining and dynamically updating a route and driving style for passenger comfort,” United States Patent, 2021.
-
C. Diels, “Will autonomous vehicles make us sick?,” in Ergonomics and Human Factors, Jan. 2014.
-
Y. Zheng, B. Shyrokau, and T. Keviczky, “3DOP: comfort-oriented motion planning for automated vehicles with active suspensions,” in 2022 IEEE Intelligent Vehicles Symposium (IV), pp. 390–395, Jun. 2022, https://doi.org/10.1109/iv51971.2022.9827152
-
Chen Tang, “Research on electromagnetic actuator for vehicle active suspension,” Zhejiang University of Technology.
-
Fangmin Mao, “Research on joint control of active suspension and braking to reduce the incidence rate of carsickness,” Zhejiang University of Technology.
-
Qiming Wang, “Research and active control of anti-motion sickness “Suspension-Seat-body” system,” Zhejiang University of Technology, 2020.
-
Z. Gao, X. Xia, Y. Liao, D. Ning, and H. Du, “Optimization of electrically interconnected suspension for vibration control,” in 2021 IEEE International Intelligent Transportation Systems Conference (ITSC), pp. 3890–3895, Sep. 2021, https://doi.org/10.1109/itsc48978.2021.9564470
-
X. M. Hu and W. L. Li, “Research on the fuzzy control for vehicle semi-active suspension,” Advanced Materials Research, Vol. 683, pp. 716–719, Apr. 2013, https://doi.org/10.4028/www.scientific.net/amr.683.716
-
E. Lenz, P. Hedrich, and P. F. Pelz, “Aktive luftfederung – modellierung, regelung und hardware-in-the-loop-experimente,” Forschung im Ingenieurwesen, Vol. 82, No. 3, pp. 171–185, Apr. 2018, https://doi.org/10.1007/s10010-018-0272-2
-
A. Baz, “Non-reciprocal piezoelectric metamaterials with tunable mode shapes,” Frontiers in Mechanical Engineering, Vol. 8, p. 1042727, Nov. 2022, https://doi.org/10.3389/fmech.2022.1042727
-
D. M. Mead, “Wave propagation in continuous periodic structures: research contributions from Southampton, 1964-1995,” Journal of Sound and Vibration, Vol. 190, No. 3, pp. 495–524, Feb. 1996, https://doi.org/10.1006/jsvi.1996.0076
-
Z. Li, “Jaguar Land Rover is committed to developing new technologies,” Automotive and Parts, Vol. 1249, No. 3, pp. 31–33, 2019.
-
S. Salter, C. Diels, P. Herriotts, S. Kanarachos, and D. Thake, “Motion sickness in automated vehicles with forward and rearward facing seating orientations,” Applied Ergonomics, Vol. 78, pp. 54–61, Jul. 2019, https://doi.org/10.1016/j.apergo.2019.02.001
-
Li Jian, “Sitting direction change can prevent motion sickness,” Fujian Agricultural Machinery, Vol. 4, 2005.
About this article
This research was funded by National Natural Science Foundation of China in 2021, grant number: 62073298; Key R&D projects in Henan Province, grant number: 232102221036; Key Scientific Research Projects of Doctoral Fund of Zhengzhou University of light industry, grant number: 2019BSJJ002. Henan Foreign Scientist Studio under Grant GZS2023011. Key Research and Development Projects of Henan Province in 2022: 221111240200. Major Science and Technology Projects of Henan Province in 2022: 221100220200.
The datasets generated during and/or analyzed during the current study are available from the corresponding author on reasonable request.
Zhijun Fu was responsible for the methodology organization; Jinliang Wu was responsible for the manuscript writing; Yuming Yin is responsible for creative development and supervision; Zhigang Zhang is responsible for the refinement of the manuscript.
The authors declare that they have no conflict of interest.