Abstract
In this paper, the three dimensional LES method was used to generate a typical turbulent wind field. Three streamlined box girders with three different aspect ratios were adopted to investigate the effects of aspect ratios on the force coefficients and flow separation characteristics with different angles of attack. The results show that the aspect ratio has significant effects on the force coefficients of the streamlined box girder, and the larger the aspect ratio is, the larger the lift coefficient is. For the flow separation characteristics, as the aspect ratio increases, the spacing of wake vortex is shortened and the wake vortex dissipation rate is accelerated.
1. Introduction
The optimization of the main girder of a bridge can effectively improve the aerodynamic performance of the bridge. For rectangular cross-section structures without wind nozzles, their aerodynamic characteristics and wake characteristics depend largely on the flow characteristics of the separated flow from the leading edge [1-5]. Usually, the aerodynamic characteristics of rectangular structures can be categorized into three states: complete separation, intermittent reattachment, and complete reattachment based on their width-to-height ratios , where and are the width and height of the rectangular section [6-8]. The results show that when 2.8, the separated flow exceeds the entire structure body and forms a strong vortex shedding in the near wake region, resulting in the structure’s large pulsation lift coefficient (). When 2.8 < 6~8, the separation flow undergoes intermittent reattachment on the sides of the structure. With a further increase in , the separated flow completely attaches to the side surfaces and separates again at the trailing edge of the structure, and the vortex shedding of the wake gradually diminishes in the state of complete reattachment. Therefore, the aspect ratio of the structure in the wind field is an important factor affecting the distribution of the flow field around the structure and the aerodynamic characteristics of the structure, and many studies have been carried out in this area.
The design of the main girder aerodynamic profile is one of the effective measures to control the wind-induced vibration of bridges, and the aspect ratio, as one of the important parameters of the main girder aerodynamic profile, is of great significance to be studied. In this paper, the effects of aspect ratios on the force coefficients, and flow separation characteristics of streamlined box girders are investigated by using 3D-LES methods.
2. Numerical validation
The formation of aerodynamic forces is largely caused by the flow separation and reattachment of fluids, which are very sensitive to the aspect ratio, turbulence intensity, and Reynolds number of streamlined box girders [9-11]. As aspect ratio is an important parameter of the main girder section profile, and main girders with different aspect ratios will cause different wind-induced vibrations in bridges, the study of aerodynamic characteristics of streamlined box girders with different aspect ratios under the action of turbulent wind is of great significance in the field of wind resistance of bridges.
2.1. Numerical method
The aspect ratio of large-span streamlined box girders usually ranges from 5.3 to 11.6, the streamlined box girders with aspect ratios of 7.5, 9.3, and 12.7 were selected to investigate the effects aspect ratios on the force coefficients and three-dimensional flow separation characteristics of streamlined box girders in turbulent field. The dimensions of the model are shown in Fig. 1.
Fig. 1Segmental models with different aspect ratios (Unit: mm)
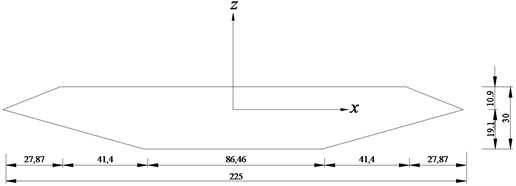
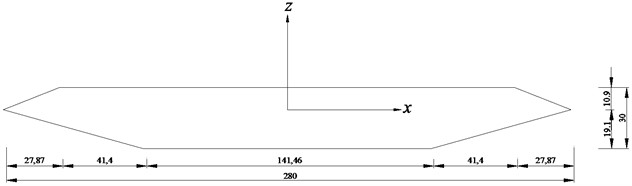
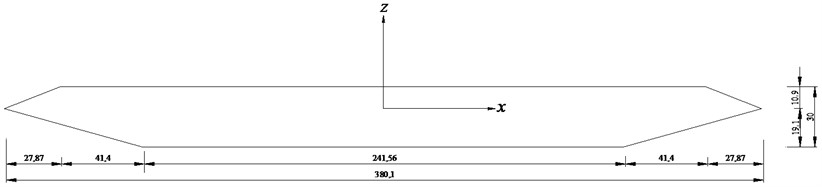
Numerical simulations are carried out using three-dimensional large-eddy simulations (3D LES) with the SGS model. The computational domain takes into consideration 6 in front, 12 behind, and 6 above/under the surfaces to ensure domain independence. Fig. 2 shows the computational domain and mesh distribution. The dimensions of the computational domain in the , , and directions are 19 × 12 × 3. The computational blocking rate is 0.04 %, which can ensure the independence of the computational domain [12, 13]. The boundary conditions of the computational domain are set as follows: the surface of the main beam is a no-slip wall boundary, the entrance is a velocity-inlet boundary, the exit is an outflow boundary, and the symmetry boundary is used on the top, and bottom, and sides of the computational domain [14, 15].
Fig. 2Details of meshing of the box girder with aspect ratios of 9.3
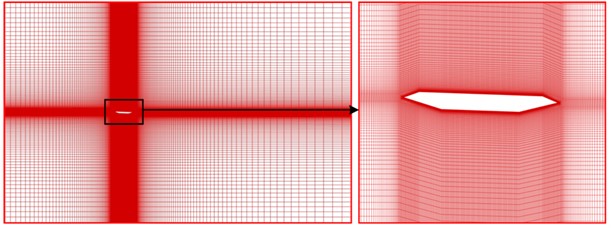
2.2. Validation
As shown in Fig. 3, the numerical results are validated by the wind tunnel tests in uniform flow. The experimental tests are carried out in a multiple-fan active control wind tunnel at Tongji University, Shanghai, China. It can be found that the lift coefficients obtained by experimental tests and numerical simulations agree well.
Fig. 3Comparisons of lift coefficients obtained by experimental tests and numerical simulations
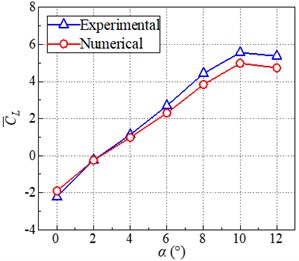
3. Results discussion
3.1. Effect of aspect ratio on lift coefficients
The LES method was adapted to simulate the turbulent field -2, of which the turbulent intensity is 7.3 %, turbulent integral scale is 0.5 m. And the angle of attack was set to ranges from 0° to 12° with an interval of 2°. The details of the numerical simulations are shown in Table 1.
Table 1Cases of box girders with different aspect ratios
Condition No. | Aspect ratio | Angle of attack (°) | Flow field characteristics |
B-1 | 7.5 | 0, 2, 4, 6, 8, 10, 12 | -2 ( 7.34%, 0.525 m) |
B-2 | 9.3 | 0, 2, 4, 6, 8, 10, 12 | |
B-3 | 12.7 | 0, 2, 4, 6, 8, 10, 12 |
Fig. 4 shows the effect of oscillating amplitude and frequency on the mean velocity distributions around the wake in different sinusoidal oscillating flows. Due to the interference of the box girder, the downstream mean velocity of the wake in the near-wake region ( 0.5) is significantly smaller than the mean incoming flow. As the flow distance increases, the mean downstream velocity gradually increases to the mean oncoming flow ( 8 m/s). The influences of oscillating frequency and amplitude on the changing trend can be ignored.
Fig. 4Effect of the aspect ratios on the aerodynamic coefficients
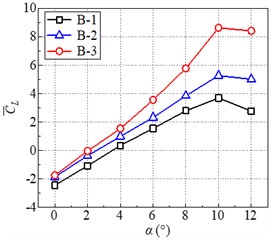
a) The effect of frequency
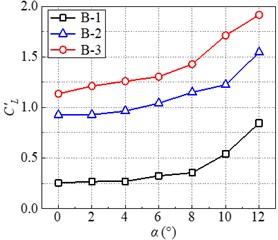
b) The effect of amplitude
3.2. Effect of aspect ratios on flow separation characteristics
To investigate the effect of aspect ratio on the streamline distribution of the flow field around the main girder, Fig. 5 shows the streamline distribution of the main girder with different aspect ratios in a turbulent wind field. The picture shows that the aspect ratio of the main beam gradually increases from left to right, and the angle of attack gradually increases from top to bottom.
Fig. 5Streamlines distributions of the box girder with different aspect ratios
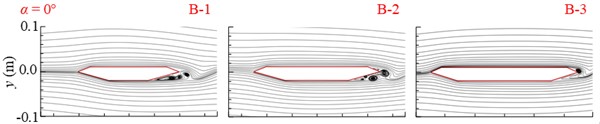
a) 0°
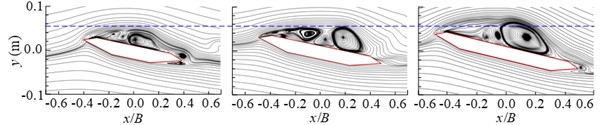
b) 12°
From the figure, it can be seen that, because in the case of small angle of attack 0°, the larger the aspect ratio of the main beam, the better the streamline characteristics of the main beam, the smaller the effect on the fluid, all the main beams of the upper and lower surfaces of the flow separation phenomenon does not occur, but only in the leeward side of the main beam appeared in the vortex, and the vortex of the leeward side of the main beam is significantly reduced in the case of the B-3 working conditions. The case of a large angle of attack (12°) is different, because the height of the flow separation point and the windward area of the main girder are significantly increased in the case of a large angle of attack, so the separation bubble on the upper surface of the main girder becomes thicker gradually with the increase of the width-to-height ratio. Therefore, in the case of a large angle of attack, the increase in aspect ratio will make the separation bubble on the upper surface of the main beam larger.
To investigate the effect of aspect ratio on the wake shedding pattern of the main beams, Fig. 6 illustrates the distribution of wake vorticity for the main beams of conditions B-1, B-2, and B-3 for the angle of attack ranging from 0° to 12°. When 0°, the wake streams of the main beams for all the working conditions show a clear phenomenon of alternating shedding of positive and negative vortices. It can be observed that the width of the wake streams of the main beams from working conditions B-1 to B-3 becomes narrower. In all the cases, the wake of the main beam dissipates around 0.4 m. The width of the main beam is gradually increasing, so the length of the wake shortens as increases. Therefore, in the case of a small angle of attack, the vortex-shedding phenomenon of the wake stream of the main beam will gradually weaken with the increase of the width-to-height ratio. When increases to 12°, "2S" vortices reappear on the upper and lower surfaces of the main beam, and the distance between positive and negative vortices increases. As the aspect ratio increases, the distance between the positive and negative vortex generation points becomes longer due to the widening of the main beam, increasing the phase difference between the positive and negative vortices in the wake stream.
Fig. 6Vertex shedding of the box girder with different aspect ratios
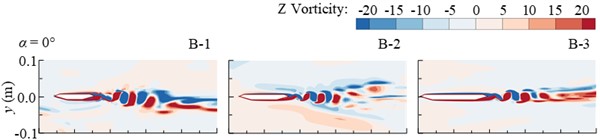
a) 0°
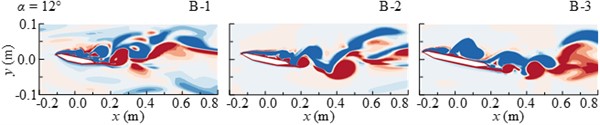
b) 12°
4. Conclusions
In this paper, three streamlined box girders were adapted to investigate the influence of aspect ratios on the force coefficients and flow separation characteristics in turbulent flow field. The main conclusions are as follows:
1) The aspect ratio has an obvious influence on the force coefficients of the streamlined box girder. The larger the aspect ratio is, the larger the lift force is.
2) The aspect ratio has significant effects on the flow separation characteristics. At angle of attack 0°, none of the main girders experienced obvious flow separation. When the angle of attack increases to 12°, significant flow separation occurs around all three main girders, and the increase in aspect ratio will make the separation bubble larger, which in turn affects the force on the main girder.
3) As the aspect ratio increases, the spacing of wake vortex is shortened and the wake vortex dissipation rate is accelerated.
References
-
F. Nagao, H. Utsunomiya, T. Oryu, and S. Manabe, “Aerodynamic efficiency of triangular fairing on box girder bridge,” Journal of Wind Engineering and Industrial Aerodynamics, Vol. 49, No. 1-3, pp. 565–574, Dec. 1993, https://doi.org/10.1016/0167-6105(93)90050-x
-
A. Larsen and A. Wall, “Shaping of bridge box girders to avoid vortex shedding response,” Journal of Wind Engineering and Industrial Aerodynamics, Vol. 104-106, pp. 159–165, May 2012, https://doi.org/10.1016/j.jweia.2012.04.018
-
B. Wu, J. Zhou, J. Xin, H. Zhang, L. Zhang, and X. Yang, “Aerodynamic forces on a bluff cylinder in sinusoidal streamwise winds with different angles of attack,” Buildings, Vol. 12, No. 7, p. 1033, Jul. 2022, https://doi.org/10.3390/buildings12071033
-
M. N. Haque, H. Katsuchi, H. Yamada, and M. Nishio, “Investigation of edge fairing shaping effects on aerodynamic response of long-span bridge deck by unsteady RANS,” Archives of Civil and Mechanical Engineering, Vol. 16, No. 4, pp. 888–900, Sep. 2016, https://doi.org/10.1016/j.acme.2016.06.007
-
H. Li, L. Zhang, B. Wu, Y. Yang, and Z. Xiao, “Investigation of the 2D aerodynamic admittances of a closed-box girder in sinusoidal flow field,” KSCE Journal of Civil Engineering, Vol. 26, No. 3, pp. 1267–1281, Mar. 2022, https://doi.org/10.1007/s12205-021-0610-z
-
R. Parker and M. C. Welsh, “Effects of sound on flow separation from blunt flat plates,” International Journal of Heat and Fluid Flow, Vol. 4, No. 2, pp. 113–127, Jun. 1983, https://doi.org/10.1016/0142-727x(83)90014-0
-
K. Shimada and T. Ishihara, “Application of a modified k-ε model to the prediction of aerodynamic characteristics of rectangular cross-section cylinders,” Journal of Fluids and Structures, Vol. 16, No. 4, pp. 465–485, May 2002, https://doi.org/10.1006/jfls.2001.0433
-
B. Wu, J. Zhou, J. Xin, H. Zhang, and F. Wu, “A numerical-experimental framework to separate the effects of different turbulence components on the buffeting forces of bluff-body structures,” KSCE Journal of Civil Engineering, Vol. 27, No. 8, pp. 3386–3402, Aug. 2023, https://doi.org/10.1007/s12205-023-0675-y
-
X. He, H. Li, H. Wang, D. Fang, and M. Liu, “Effects of geometrical parameters on the aerodynamic characteristics of a streamlined flat box girder,” Journal of Wind Engineering and Industrial Aerodynamics, Vol. 170, pp. 56–67, Nov. 2017, https://doi.org/10.1016/j.jweia.2017.08.009
-
B. Wu, J. Zhou, S. Li, J. Xin, H. Zhang, and X. Yang, “Combining active and passive wind tunnel tests to determine the aerodynamic admittances of a bridge girder,” Journal of Wind Engineering and Industrial Aerodynamics, Vol. 231, p. 105180, Dec. 2022, https://doi.org/10.1016/j.jweia.2022.105180
-
Y. Gang, Y. Yang, W. Bo, L. Lianjie, and Z. Liangliang, “Aerodynamic admittance influence on buffeting performance of suspension bridge with streamlined deck,” Journal of Vibroengineering, Vol. 21, No. 1, pp. 198–214, Feb. 2019, https://doi.org/10.21595/jve.2018.19681
-
F. Baetke, H. Werner, and H. Wengle, “Numerical simulation of turbulent flow over surface-mounted obstacles with sharp edges and corners,” Journal of Wind Engineering and Industrial Aerodynamics, Vol. 35, pp. 129–147, Jan. 1990, https://doi.org/10.1016/0167-6105(90)90213-v
-
L. Liu, L. Zhang, B. Wu, and B. Chen, “Numerical and experimental studies on grid-generated turbulence in wind tunnel,” Journal of Engineering Science and Technology Review, Vol. 10, No. 3, pp. 159–169, Jul. 2017, https://doi.org/10.25103/jestr.103.21
-
H. Li, L. Zhang, B. Wu, and Z. Xiao, “Effects of streamwise sinusoidal flow on the aerodynamic characteristics of a box girder,” Applied Sciences, Vol. 12, No. 11, p. 5341, May 2022, https://doi.org/10.3390/app12115341
-
H.-H. Li, L.-L. Zhang, B. Wu, Z.-J. Ni, and Y. Yang, “Effects of the angle of attack on the aerodynamic characteristics of a streamlined box girder,” Advances in Structural Engineering, Vol. 24, No. 10, pp. 2090–2104, Jul. 2021, https://doi.org/10.1177/1369433221992490
About this article
This study was funded by the Science and Technology Research Program of Chongqing Municipal Education Commission under the grant number KJZD-M202302501, KJQN-202002504.
The datasets generated during and/or analyzed during the current study are available from the corresponding author on reasonable request.
The authors declare that they have no conflict of interest.