Abstract
To reveal the viscosity-enhanced mechanism of super-viscous heavy oil and improve the recovery rate of super-viscous heavy oil, the four components, elemental composition, rheological properties, and effects of asphaltenes and resin on the viscosity of super-viscous heavy oil from well TH12434 in Tahe Oilfield, China have been analyzed from macro and microscopic perspectives by Anton Paar rotational rheometer, gas chromatography-mass spectrometry and scanning cryo-EM to solve the problems of poor fluidity and high asphaltene content. The experimental results showed that in the temperature range of 40-100°C, the viscosity of super-viscous heavy oil decreases sharply from 352000 mPa∙s to 1620 mPa∙s, and the super-viscous heavy oil exhibits clear thermo-sensitivity. With 100°C and shear rate ranging from 0-800 s-1, the viscosity of super-viscous heavy oil decreases sharply from 45000 mPa∙s to 956 mPa∙s, and the oil sample shows typical pseudoplasticity. The baseline of super-viscous heavy oil analysis by gas chromatography shows too high, and more than 80 % of super-viscous heavy oil compounds have a matching degree of less than 70 % with standard compounds, indicating that the super-viscous heavy oil had poor heterogeneity and many impurities. It is observed by scanning cryo-EM that the micromorphology of super-viscous heavy oil is large granular, strong continuity, asphaltene micromorphology presents an obvious layered structure, the layer spacing is 637.7 nm, and its asphaltene molecules form an order-like or crystal-like association structure through several unit sheet layers, resulting in high viscosity of super-viscous heavy oil. Based on the analysis results of the influencing factors of the viscosity of super-viscous heavy oil, a theoretical basis for the selection of viscosity reduction technology for super-viscous heavy oil the efficient exploitation in Tahe Oilfield, China could be provided.
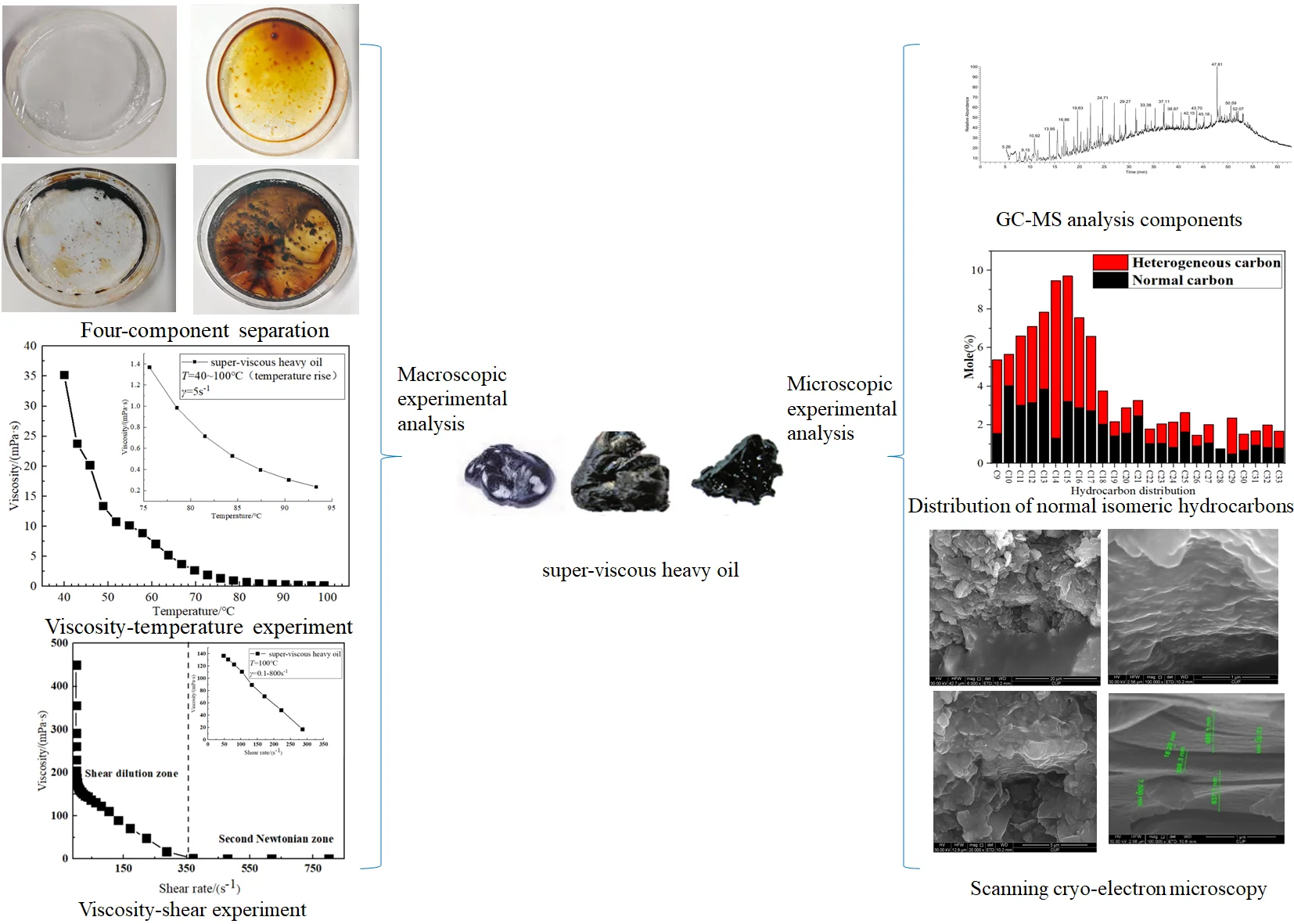
Highlights
- The macroscopic and microscopic viscosity influencing factors of super-viscous heavy oil in Tahe oil fields, China have been analyzed.
- The mechanism of viscosity induced by super-viscous heavy oil has been revealed by macroscopic four-component analysis, viscosity-temperature and viscosity-shear experiments, microscopic gas chromatography and scanning electron microscope analysis.
- The viscosity-temperature and viscosity-shear curves of super-viscous heavy oil have been obtained and the linear viscosity shear fitting curve equation of super heavy oil has been obtained.
1. Introduction
Heavy oil resources account for about 70 % of the total oil. Due to the increasing global energy demand and the limited supply of conventional crude oil production chain, heavy oil exploitation will occupy a major position in future oil and gas development [1]. Among them, Tahe Oilfield in China is a typical heavy oil reservoir, which is located on the northern margin of the Tarim Basin. The reservoir type is fracture-vuggy carbonate super-deep and super-heavy oil reservoir, and the burial depth of heavy oil is 5500 m-7000 m, which is the largest buried heavy oil reservoir found in China so far. Tahe Oilfield is characterized by deep reservoir burial, high formation pressure, high sulfur content, poor fluidity, and high asphaltene content. Therefore, it is a breakthrough to solve the problem of heavy oil exploitation and transportation to study the causes of high viscosity and poor fluidity in the Tahe oilfield.
At present, the research results obtained at home and abroad show that heavy oil has a high content of heavy components, asphaltene and gum are the main reasons for the high viscosity of heavy oil. Asphaltene is composed of some polymer hydrocarbons and polar heteroatoms, and its molecular structure and relative molecular mass are uncertain. The molecular structure is very complex, mainly composed of an aromatic ring, an alkyl side chain, and a cycloalkane system. The presence of heteroatoms (N, S, and O) and heavy metals (V, Ni) makes asphaltene the most polar part of petroleum [2-4]. Some scholars have found that the gum contains a large number of phenolic and ether compounds with aromatic structures, so it has a strong polarity, and its aromatic property is smaller than asphaltene, and the effect of interleaving and chemical bonding between the gum will lead to its polymerization, thereby increasing the viscosity of heavy oil [5, 6]. At the same time, the colloidal system in heavy oil is generally unbalanced, which is not enough to completely wrap asphaltene and evenly disperse it in saturated hydrocarbons and aromatic hydrocarbons. Under the action of hydrogen bonding, asphaltene molecules form complex aggregates by stacking side to side and side to side, and the colloidal molecules are superimposed on the surface of asphaltene to form aggregate particles. The particles connect to form a more intricate aggregation structure, which makes heavy oil with high viscosity and difficult to exploit [7]. In addition, at low temperatures, paraffin in heavy oil will precipitate in the form of crystallization, and the presence of asphaltene molecules provides a nucleation center for wax crystals, so wax molecules are more likely to precipitate and grow on the surface of asphaltene. Asphaltene molecules increase the number of wax molecular connection points [8], and it is easy to form a network structure, which greatly reduces the fluidity of heavy oil and increases the viscosity of heavy oil. However, due to the extremely complex composition of super-viscous heavy oil, the properties of super-viscous heavy oil vary greatly in different oil fields and different blocks. The above studies have analyzed the adhesion mechanism of asphaltene, gum, and paraffin-associated aggregates on heavy oil in specific heavy oil fields. However, for the super-viscous heavy oil in the Tahe oilfield, there is still no corresponding analysis on the influence of viscosity factors. It is necessary to carry out in-depth research on the macroscopic and microscopic viscosity reduction mechanism of super-viscous heavy oil. Therefore, to improve the exploitation efficiency of super-viscous heavy oil in Tahe Oilfield and realize the high-efficiency viscosity reduction of super-viscous heavy oil, taking the production and export of super-viscous heavy oil from well TH12434 in Tahe Oilfield as an example, the influence mechanism of macro four components, temperature, shear rate, microscopic asphaltene and gum on the viscosity of super-viscous heavy oil is analyzed, which has great industrial guiding significance for solving the problem of efficient exploitation of super-viscous heavy oil in Tahe oilfield.
2. Experimental equipment and method
2.1. Experimental materials and instruments
Super-viscous heavy oil samples are from well TH12434, Tahe Oilfield, which show a solid-state room temperature and atmospheric pressure; The reagents used in the separation of four components of super heavy oil are from Sinopharm Chemical Reagent Beijing Co., LTD.
The viscosity and shear rate of super heavy oil are tested using the MCR 302 laboratory rheometer (Anton Paar, Austria). The super-viscous heavy oil is analyzed by SQI chromatomass spectrometry (THERMOFISHER, America). Phenmo pro X scanning electron microscope (SEM) is used to observe the morphology of super-viscous heavy oil.
2.2. Experimental method
1. Four-component separation: According to NB SH/T 0509-2010 Method for Determination of four Components of Petroleum asphalt, the four components of super-viscous heavy oil in Tahe oilfield were determined.
2. GC-MS analysis of heavy oil components: The characteristic molecular markers of super-viscous heavy oil were analyzed by Thermo Fisher SQI chromatograph-mass spectrometer.
3. Viscosity temperature performance test of super-viscous heavy oil: The viscosity-temperature of Tahe ultra-heavy oil was measured by Anton Par MCR302 rotary rheometer plate rotor system.
4. Viscosity shear performance test of super-viscous heavy oil: The viscous shear test of Tahe ultra-heavy oil was carried out by using Anton Par MCR302 rotating rheometer coaxial cylinder system.
5. Scanning cryo-electron microscopy: Phenmo pro-X scanning cryo-electron microscope (SEM) produced by Thermo Scientific was used to observe the microscopic morphology of super-viscous heavy oil and asphaltene in Tahe oilfield.
3. Results and discussion
3.1. Composition analysis of super-viscous heavy oil in Tahe Oilfield
Super-viscous heavy oil has good fluidity under high temperature (120 ℃-150 ℃) and high pressure (1 MPa) in deep formation 5732 m, though during dring from near surface to pipeline has high viscosity, poor fluidity and easy solidification. In order to solve this problem, factors affecting viscosity of ultra-heavy oil under normal pressure and 40-60 ℃ are considered. The specific analysis results of ultra-heavy oil components in well TH12434 of Tahe Oilfield are shown in Table 1. It can be seen from the table that the asphaltene content of ultra-heavy oil is as high as 24.08 %, and the content of asphaltene and gum components accounts for almost half of the total components, which is also the key factor causing the extremely high viscosity of ultra-heavy oil [9]. Among them, the physical picture of super-viscous heavy oil separation is shown in Fig. 1.
Table 1The content of each component of super-viscous heavy oil
Heavy oil component (wt%) | |||
Saturates | Aromatics | Asphaltenes | Resins |
20.55 % | 35.17 % | 24.08 % | 20.20 % |
In addition, the ultra-heavy oil of well TH12434 has a high sand content, and the analysis determined that 1.1522 grams of oil sample contained 0.2541 grams of sand (sand content 22 %). It shows that Tahe heavy oil contains sediment and other impurities, which makes it difficult to separate components and treat heavy oil.
Fig. 1Physical picture of component separation of ultra-heavy oil
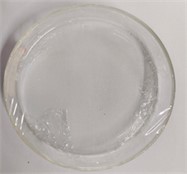
a) Saturates
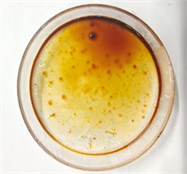
b) Aromatics
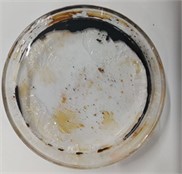
c) Resins
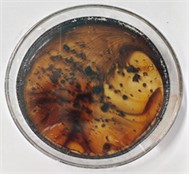
d) Asphaltenes
3.2. Gas chromatographic analysis of heavy oil
Based on Thermo FisherSQI chromatograms and mass spectrometers, GC-MS chromatograms and mass spectrometry were carried out on the super-viscous heavy oil of well TH12434 in Tahe Oilfield, and the percentage distribution of the super-viscous heavy oil was obtained, as shown in Fig. 2.
Fig. 2GC-MS experimental results of ultra heavy oil
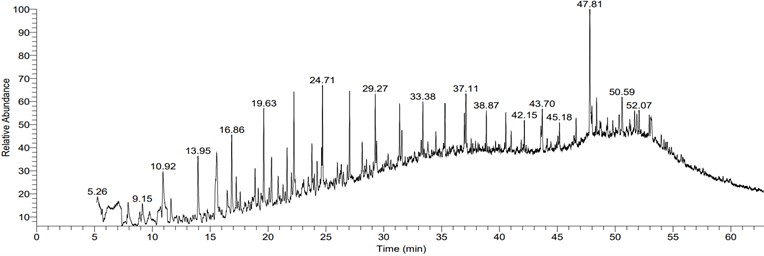
The main peak is the normal saturated hydrocarbon, and the smaller peaks between the normal alkanes are mostly isomeric alkanes with the same carbon number. It can be seen from the analysis of Fig. 2 that the content of normal paraffin in super-viscous heavy oil is small, and the components are mainly iso-alkanes and a small amount of aromatic hydrocarbons. The baseline of column percentage is too high, which may be caused by the fact that aromatic hydrocarbons or cycloalkanes contain partially branched chains (including branched-chain structures such as carboxylic acids). Meanwhile, the matching degree between more than 80 % of super-viscous heavy oil compounds and standard compounds is lower than 70 %. It shows that the composition of heavy oil samples is poor inhomogeneity, and there are some complex branch chains and heteroatoms.
Fig. 3 shows the distribution of n-isomeric hydrocarbons in gas chromatography of super-viscous heavy oil. Since the chromatographic column temperature of the chromatograph can only reach 400 ℃, only the component ratio of N-isomeric alkanes in the range C9-C33 was obtained and analyzed. As can be seen from Fig. 3, the proportion of iso-alkanes is more than 50 %, indicating that there is a complex chemical chain reconstruction process during the formation of super-viscous heavy oil. Nearly 51.7 % of the hydrocarbons in super-viscous heavy oil are concentrated in the light component (≤ C15), and only 6.8% in the heavy component (≥ C30). The difference in hydrocarbon distribution in Fig. 3 shows that the hydrocarbons in super-viscous heavy oil have a high isomeric degree between C9 and C33, while the hydrocarbons in the light components C10 and C17 show a normal distribution.
The high content of isomeric hydrocarbon, complex branch chain, and heteroatom in super-viscous heavy oil reflect the complexity and variety of its components, which makes it difficult to treat and develop super-viscous heavy oil.
Fig. 3Distribution of normal isomeric hydrocarbons in ultra-heavy oil
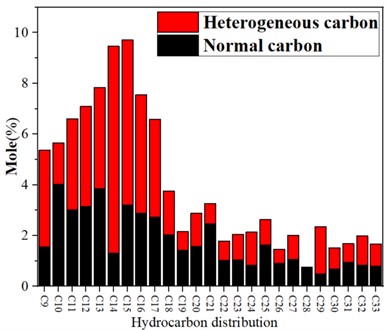
3.3. Effect of temperature on rheological properties of heavy oil
Fig. 4 shows the viscosity-temperature curve of super-viscous heavy oil in well TH12434 of Tahe Oilfield in the temperature range of 40-100 ℃. The oil sample has a viscosity of 108,000 mPa∙s at 50 ℃ and 1620 mPa∙s at 100 ℃.
Fig. 4Viscosity temperature curve of super-viscous heavy oil
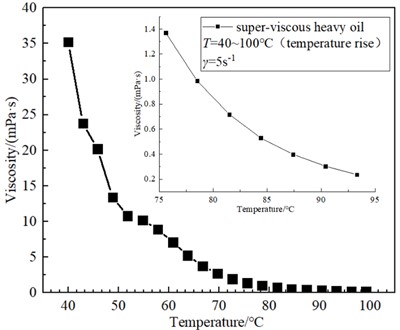
It can be seen from Fig. 4 that with the temperature rising from 40 ℃ to 90 ℃, the viscosity of ultra-heavy oil decreases rapidly, and it is extremely sensitive to temperature. This is because temperature has a drastic effect on the viscosity and chemical chain of heavy components (gum and asphaltene). Combined with gas chromatographic analysis, it is found that part of the branch chain of asphaltene (containing branch chain structure such as carboxylic acid) is easily broken and damaged by high temperature, resulting in chemical chain fracture of asphaltene, which shows that the viscosity of ultra-heavy oil decreases sharply at high temperature.
3.4. Effect of shear rate on rheological properties of heavy oil
Fig. 5 shows the viscosity curve of super-viscous heavy oil from well TH12434 in Tahe Oilfield in the range of 0-800 s-1 at 100 ℃. According to the analysis of Fig. 5, the viscosity of super-viscous heavy oil decreases rapidly with the increase of shear rate, and its shear dilution zone ranges from 50-350 s-1 and reaches the second Newton zone when the shear rate is 350 s-1. The viscosity does not change as the shear rate increases.
Fig. 5Viscoshear curve of super-viscous heavy oil
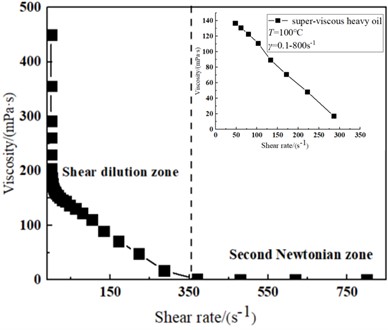
The results of the viscous shear test show that the oil sample exhibits typical pseudoplasticity (shear dilution). This is because asphaltene and gum form a cross-linked spatial network structure with the precipitated wax crystals through the micellar structure composed of side stacking or side stacking [10-12]. At high temperatures, with the increase of shear rate, the damage rate of the cross-linked spatial network structure is greater than the reconstruction rate, resulting in the decrease of viscosity and the appearance of pseudoplasticity.
To characterize the shear dilution of non-Newtonian fluids, a power-law fluid model is used, as shown in Eq. (1):
where is viscosity; is the consistency coefficient; is the shear rate; is the fluidity index.
Fig. 6Linear fitting diagram of viscosity shear curve for super-viscous heavy oil
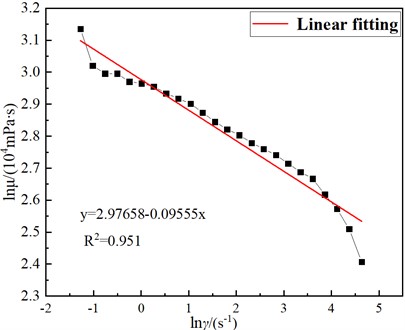
Fig. 6 shows the linear fitting process of Eq. (1), and the fitting calculation results are shown in Table 2. The experimental results show that the power law fluid model has high fitting accuracy for Tahe super-viscous heavy oil, and the oil sample has strong pseudoelasticity.
Table 2Linear fitting equation for ultra-heavy oil
℃ | Fitting equation | Coefficient of viscosity | R2 |
100 | 0.90455 | 0.951 |
3.5. Influence of micromorphology of asphaltene and gum on the viscosity of heavy oil
Scanning cryo-electron microscopy was used to observe the microscopic morphology of super-viscous heavy oil and its asphaltene in well TH12434 of Tahe Oilfield, as shown in Fig. 7. It can be seen from Fig. 7(a) and (b) that super-heavy oil exists in a continuous state and more asphaltene particles are dispersed. In Fig. 7(a), it can be observed that when the SEM is enlarged by 6000 times, the aggregate of asphaltene has a strong granular feeling and is large granular. Fig. 7(d) shows the microscopic morphology of asphaltene at 100,000 times magnification. It can be seen that asphaltene presents an obvious layered structure with a tiny layer spacing of 637.7 nm.
The reason for the layered structure of asphaltene is that the asphaltene molecule is composed of several unit sheets (aromatic sheets), which are associated together through intramolecular or intermolecular interaction forces to form a partially ordered crystalline asphaltene sheet structure, and further associated through intermolecular hydrogen bonds, conjugated forces and other chemical bonds to form a larger polymeric molecular structure. This results in a high viscosity of super-viscous heavy oil.
Fig. 7Micromorphology of super-viscous heavy oil and asphaltene scanning electron microscopy
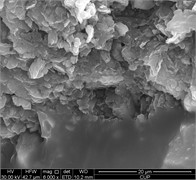
a) 6000 times
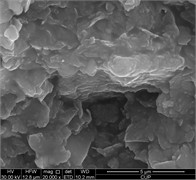
b) 20000 times
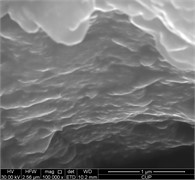
c) 40000 times
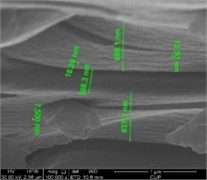
d) 100000 times
4. Conclusions
1) The content of asphaltene and colloid in the super-viscous heavy oil of well TH12434 in Tahe Oilfield is as high as 24.08 % and 20.2 %, and the high content of colloid asphaltene is the key reason for the high viscosity of the super-viscous heavy oil.
2) The content of normal paraffin in super-viscous heavy oil is small, the proportion of isomeric paraffin is more than 50 %, the percentage of chromatograms is high, and the matching degree between more than 80 % of super-viscous heavy oil compounds and standard compounds is less than 70 %, indicating that the composition of heavy oil samples has poor homogeneity, complex branch chains, and heteroatoms.
3) Super-viscous heavy oil has strong temperature sensitivity, and the viscosity decreases rapidly with the temperature rising to 100 ℃, decreasing by about 99 %, which is due to the chemical chain fracture of asphaltene gum caused by high temperature.
4) Under the condition of 100 ℃ and linear increase of shear rate 0-800 s-1, the viscosity of super-viscous heavy oil decreases sharply from 45000 mPa∙s to 956 mPa∙s. Under high-temperature conditions, with the increase of shear rate, the damage rate of crosslinked spatial network structure formed by asphaltene gum is greater than the reconstruction rate. The super-viscous heavy oil presents a typical pseudoelasticity.
5) Scanning cryo-electron microscopy shows that asphaltene has a layered structure with small spacing between lamellar layers. The reason for the lamellar structure of asphaltene is that asphaltene molecules are composed of several unit sheets (aromatic sheets), which are associated together through intramolecular or intermolecular interactions to form a partially ordered crystalline asphaltene lamellar structure. Further through the intermolecular hydrogen bond, conjugation force, and other chemical bonds form a larger polymer molecular structure, resulting in a high viscosity of super-viscous heavy oil.
References
-
P. Druetta, P. Raffa, and F. Picchioni, “Chemical enhanced oil recovery and the role of chemical product design,” Applied Energy, Vol. 252, p. 113480, Oct. 2019, https://doi.org/10.1016/j.apenergy.2019.113480
-
Y. M. Ganeeva, E. E. Barskaya, E. S. Okhotnikova, and T. N. Yusupova, “Features of the composition of compounds trapped in asphaltenes of oils and bitumens of the Bavly oil field,” Energy and Fuels, Vol. 35, No. 3, pp. 2493–2505, Feb. 2021, https://doi.org/10.1021/acs.energyfuels.0c03022
-
B. Schuler et al., “Overview of Asphaltene Nanostructures and Thermodynamic Applications,” Energy and Fuels, Vol. 34, No. 12, pp. 15082–15105, Dec. 2020, https://doi.org/10.1021/acs.energyfuels.0c00874
-
F. Souas, A. Safri, and A. Benmounah, “A review on the rheology of heavy crude oil for pipeline transportation,” Petroleum Research, Vol. 6, No. 2, pp. 116–136, Jun. 2021, https://doi.org/10.1016/j.ptlrs.2020.11.001
-
T. Li et al., “Resin from liaohe heavy oil: molecular structure, aggregation behavior, and effect on oil viscosity,” Energy and Fuels, Vol. 32, No. 1, pp. 306–313, Jan. 2018, https://doi.org/10.1021/acs.energyfuels.7b03279
-
J. M. Santos, A. Vetere, A. Wisniewski, M. N. Eberlin, and W. Schrader, “Modified SARA method to unravel the complexity of resin fraction(s) in crude oil,” Energy and Fuels, Vol. 34, No. 12, pp. 16006–16013, Dec. 2020, https://doi.org/10.1021/acs.energyfuels.0c02833
-
Y. Zhang, M. Siskin, M. R. Gray, C. C. Walters, and R. P. Rodgers, “Mechanisms of asphaltene aggregation: puzzles and a new hypothesis,” Energy and Fuels, Vol. 34, No. 8, pp. 9094–9107, Aug. 2020, https://doi.org/10.1021/acs.energyfuels.0c01564.
-
X. Zhang, F. Yang, B. Yao, C. Li, D. Liu, and G. Sun, “Synergistic effect of asphaltenes and octadecyl acrylate-maleic anhydride copolymers modified by aromatic pendants on the flow behavior of model waxy oils,” Fuel, Vol. 260, p. 116381, Jan. 2020, https://doi.org/10.1016/j.fuel.2019.116381
-
R. N. Bahtizin, R. M. Karimov, and B. N. Mastobaev, “The effect of high-molecular components on flow properties, depending on the structural-group and fractional oil content,” SOCAR Proceedings, pp. 42–50, Mar. 2016, https://doi.org/10.5510/ogp20160100269
-
Z. Jiang, Z. Liu, B. Fei, Z. Cai, Y. Yu, and X.E. Liu, “The pyrolysis characteristics of moso bamboo,” Journal of Analytical and Applied Pyrolysis, Vol. 94, pp. 48–52, Mar. 2012, https://doi.org/10.1016/j.jaap.2011.10.010
-
N. A. Sami, D. S. Ibrahim, and A. A. Abdulrazaq, “Investigation of non-Newtonian flow characterization and rheology of heavy crude oil,” Petroleum Science and Technology, Vol. 35, No. 9, pp. 856–862, May 2017, https://doi.org/10.1080/10916466.2017.1280505
-
R. M. Karimov, R. R. Tashbulatov, and A. R. Valeev, “About complex oil flow curve form in anomaly viscosity low temperatures and shear rates conditions,” Materials Science Forum, Vol. 1086, pp. 131–139, Apr. 2023, https://doi.org/10.4028/p-51m1q2
About this article
We would like to acknowledge support from assistance from Ufa State Petroleum Technological University and Southwest Petroleum University. This work was supported by the National Natural Science Foundation of China (No. 52004241), the Natural Science Foundation of Sichuan Province (No. 2022NSFSC1018), the Open Fund Project of SINOPEC Key Laboratory for EOR of Fractured-vuggy Reservoir (34400000-22-ZC0607-0006).
The datasets generated during and/or analyzed during the current study are available from the corresponding author on reasonable request.
Yang Chen: conceptualization, formal analysis, funding acquisition, investigation, resources, software, supervision, validation, writing – review and editing. Jin Luo: data curation, formal analysis, investigation, project administration, software, visualization, writing – original draft preparation. Meiyu Zhang: methodology, project administration. Minglan He: methodology, project administration.
The authors declare that they have no conflict of interest.