Abstract
Assistive technologies can improve greater participation in society and sociocultural experiences. A communication device for deafblind persons, called Caeski, is an assistive technology that allows independent communication between two deafblind persons or between deafblind and non-deafblind persons using vibrating keys. The aim of this study is to analyze the effects of hand-arm vibrations (HAV) emitted by Caeski to check if this will be harmful to users. The vibrations emitted by the Caeski’s keys were analyzed from data provided by a triaxial accelerometer, positioned over the fingers of a sample of seven deafblind individuals. The obtained data are within acceptable limits set by International Health and Safety standards – ISO 8041, ISO 5349-1 and European Directive 2002/44/EC – on the physical risk of vibration. Safety is part of the product development process. It was possible to demonstrate that the proposed technology is not harmful to users and can be used safely.
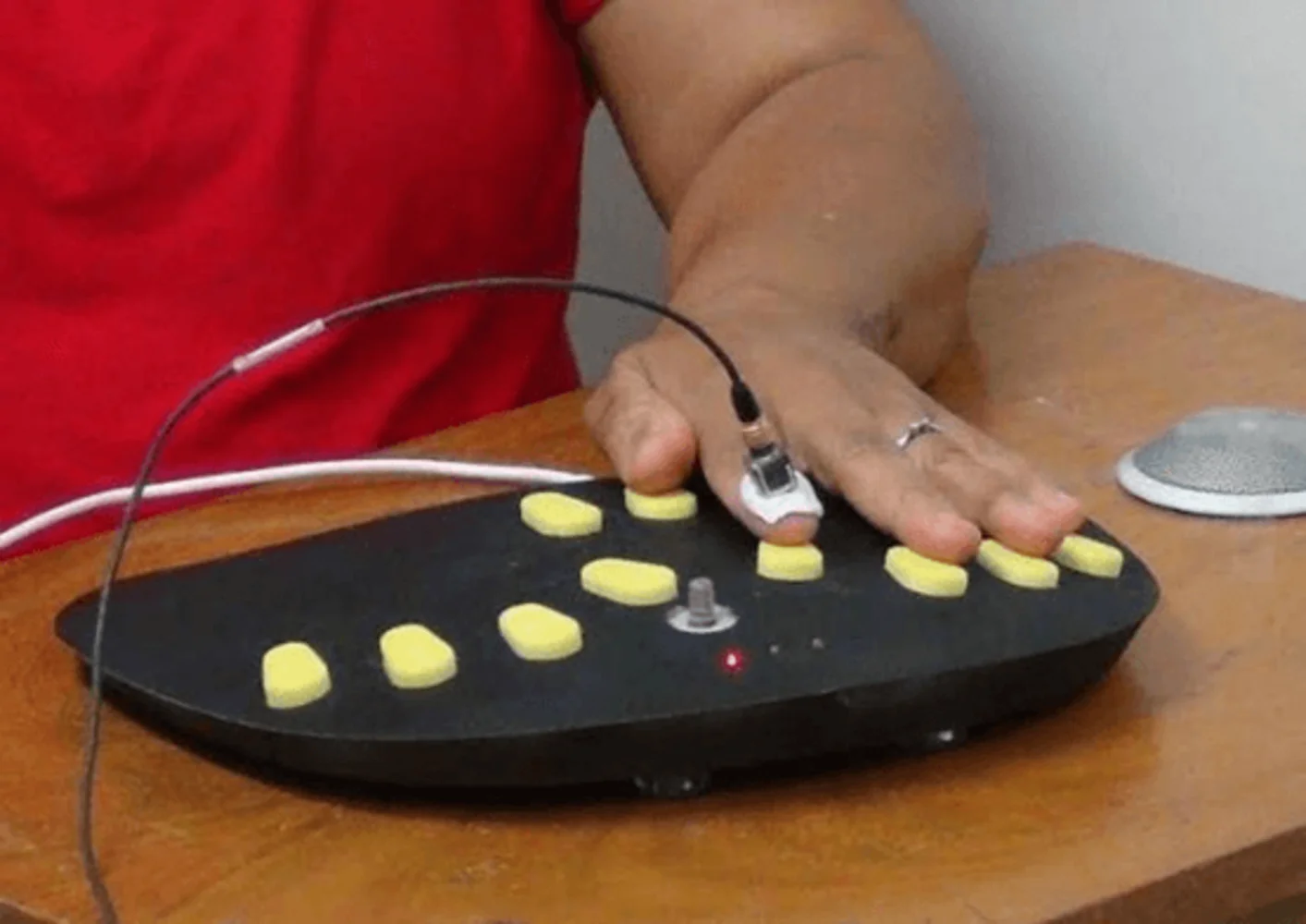
Highlights
- An analysis of hand-arm vibrations (HAV) of an assistive technology for deafblinds.
- The vibrations were measured by triaxial accelerometer positioned over the fingers.
- The assistive technology is safe as to the physical risk related to the HAV exposure.
1. Introduction
The deafblindness involves simultaneous deficits in vision and hearing, thus limiting access to a replete world of auditory and visual information. This condition can cause in children and youth deafblinds developmental and educational needs that cannot be accommodated in special education programs for children who are blind or deaf or other types of multiple disabilities. The educational challenges related to deafblindness vary based upon a student’s individual needs to ensure that person with this disability can reach their full potential [1].
The greatest difficulty for deafblind person, caused by the lack of oral communication, is the access to information. However, the desire and need to express and interact with the world make deafblind persons create different communication methods of their own. The main forms of communication for deafblind persons are: (a) Tactile sign language: with the hand in contact with the deafblind’s palm; (b) Tadoma’s method: It is formed by the hand of the deafblind person on the lips, cheek, jaw and throat of the interlocutor; (c) Handwriting: which consists of writing capital letters in the palm of the hand; (d) Extended speech: which consists of speaking clearly, near the deafblind’s ear; (e) Extended writing for visually impaired person: it is an alphabetic system that relies on writing with extended types to be perceived by the deafblind; (f) Loud talking: which is the use of a sound amplifier that helps to receive a certain message [2]. Some of these methods requires at least a small quantity of hearing or sight, what is not always possible. Therefore, other methods are necessary such as the one developed in this paper.
Around the world, various interfaces were developed to meet the communication needs to the deafblind population. A large part of these technologies was developed in glove designs [3, 4] which present a risk of disconnection due to attrition from continuous use and, also, to having small, medium and large sizes that may not cover the anthropometric variability of the hands. Other assistive resources involve the letter amplification or volume enhancement of speech synthesizers, as well requiring some degree of sight or hearing.
The Digital Age promoted innovations and advances in all aspects, shortening distances between people, especially during the COVID-19 pandemic. Thus, the inclusion of deafblind person in this scenario has become extremely important, but with safety. For the use of assertive assistive technologies and to ensure the social integration of these disabled is necessary to help communication between the deafblind person and the society in general [4]. These technological innovations provide disabled person greater autonomy, participation, and sharing of their socio-cultural experiences and broadening the exchange of this experience with other cultures [5].
The development of Caeski involved several areas of knowledge, such as electronics and integrated circuits, programming, optimization, 3D printing and design, ergonomics, pedagogy of special education and digital media, that includes the creation of a social network for deafblind persons. Thus, the use of Caeski will provide communication with self-sufficiency and freedom, overcoming the barriers that requires vision and hearing. In this way, the Caeski aims to promote digital inclusion in contexts mediated by technology promoting the effective inclusion of persons with total or partial deafblindness.
However, it is a device that emits vibrations, so it is necessary to analyze the risk of exposure to vibrations. The vibrations generated by the buttons are transmitted to the user’s fingers, which characterizes a type of vibration exposure called hand-arm vibration (HAV), which involves specific calculations and analysis for this type of vibration exposure.
Vibration can cause discomfort and harm to the human body, so the vibrational parameters must be analyzed, and their risks evaluated. Such analysis is a fundamental part of the product development process, after all, product safety cannot be neglected. In that context, this work seeks to analyze whether the use of Caeski is safe with respect to neuromusculoskeletal risks of upper limbs from exposure to vibrations.
The hand-arm vibration syndrome (HAVS) is generated by prolonged exposure to vibrations at harmful levels to the upper limbs. The vibration syndrome has adverse circulatory and neural effects in the fingers, the signs and symptoms include numbness, pain, and blanching. Called white finger syndrome, or Raynaud's phenomenon [6, 7], it refers to changes in the circulatory blood vessel or peripheral nerve disorders with loss of sensation in the extremities of the fingers. These changes can also lead to muscle aches and pain.
2. Materials and methods
Caeski consists of a keyboard interface containing vibrating buttons. Two interfaces were designed: version 1 (Fig. 1(a)) and the final version, version 2 (Fig. 1(b)), which contemplates the modifications suggested by the deafblind persons sample that participated in this project [8]. It was developed in the Bioengineering Laboratory (LabBio) of the Post-Graduate Program in Mechanical Engineering (PPGMEC) of the Universidade Federal de Minas Gerais (UFMG).
Fig. 1Versions of prototype keyboards with vibrating buttons. Source: Authors
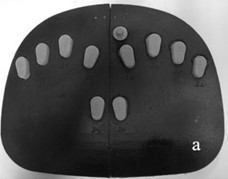
a) First version
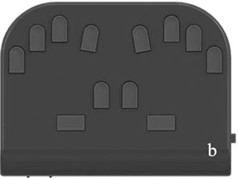
b) Final version
The combinations of these pressed buttons correspond to letters, numbers and symbols and the information reception occurs by the combined vibration of these buttons. The caller can use another Caeski if deafblind or any Android mobile device with the Caeski app installed, if non-deafblind. In both cases, communication occurs via Bluetooth.
The system has 12 buttons, each one composed of a motor DC 2.5-4V and 9000 rpm (in 4.0 V), represented by the grey keys of the device, as shown in Fig. 1. In addition to the motors and buttons, the system is composed by a microcontroller, battery and module charging for the power circuit, Bluetooth communication module and electronic components for the data sending and receiving circuit for the interface with Android mobile devices [8].
2.1. The hand-arm vibration and the determination of tolerance and action levels
Although the levels of vibration produced by Caeski are not high, it is important to consider the harmfulness of a handheld device that produces vibration from its conception and to measure such vibration levels in the prototyping phase.
The European Directive 2002/44/EC [9] establishes the value of 2.5 m/s2 as the exposure action value (EAV) to hand-arm vibration and the value of 5.0 m/s2 as the exposure limit value (ELV) for an 8 hour exposure time.
The ISO 5349-1:2001 standard [10] defines that the method of measuring exposure to vibration levels transmitted to the hand and arm shall be analyzed by weighted acceleration on the three orthogonal axes in the one-third octave range with frequencies between 6.3 Hz and 1,250 Hz. Vibration is measured by instruments connected to an acceleration transducer or accelerometer, which shall follow the specifications of ISO 8041-1: 2017 [11], ISO 5349-2: 2001 [12].
The measurements shall be made along the three orthogonal reference axes . Thus, for each of the three reference axes, the effective frequency-weighted acceleration value is obtained and from these, the total vibration value () is determined by Eq. (1) [7, 10]:
where: is total vibration acceleration value measured in , and directions; is vibration acceleration value measured in -direction at the hand; is vibration acceleration value measured in -direction at the hand; is vibration acceleration value measured in -direction at the hand.
The triaxial accelerometer must be placed with special care to respect the orientation of the coordinate axes. The vibration exposure assessment [7, 10] considers the daily exposure time , defined as the total time the hands are exposed to vibration during the performance of an activity, and is a reference time of 8 hours according to Eq. (2):
where: is daily vibration exposure related to an 8 hours period; is time of exposure to hand and arm vibration in hours or minutes; is standard time of 8 hours or 480 minutes; is total vibration acceleration value measured in , and directions, calculated according to Eq. (1):
The Daily Vibration Exposure is the total frequency-weighted amount of vibration expressed in terms of an 8-hour equivalence of daily exposures. All accelerations considered in this pattern are frequency weighted according to the weighting curve as a function of the hand response sensitivity at different frequencies, according to the weighting curve at the different frequencies present in ISO standard 8041:2017 [9].
The Standard ISO 5349-1:2001 [10] establishes daily acceleration exposure values with the expected estimate that 10 % of person exposed to hand and arm vibration may develop Raynaud’s syndrome in a given number of years () according to Eq. (3).
The mean acceleration is obtained by calculating the effective RMS value of the signals from each volunteer’s sample finger. Then, the average acceleration resulting from the three axes (, and ) was calculated and then, the resulting acceleration of the partial exposure was calculated by averaging the resulting accelerations. With this average, the standardization was made to 8 hours obtaining , as if the volunteer uses the device in a work environment performing an occupational function in the labor market. With these data, one sought to verify the total duration of exposure in years required for the occurrence of Raynaud syndrome. The IBM SPSS® Statistics software was used to average volunteers using the same device.
2.2. Experimental design and instrumentation
The invitation to participate in this study was publicized at the institutions the Educational Association for Multiple Disabilities (AHIMSA) and the National Federation of Education and Integration of the Deaf (FENEIS) that assists deafblind persons. Invitations were made through posters, emails and telephone contacts to 50 persons, among them, 30 persons expressed interest, 20 were willing to participate, however there were 5 dropouts and 8 did not fit the inclusion criteria.
In a second moment, candidates to the research sample answered an anamnesis form. The data collected refers to the full name, age, address and phone numbers, education level, history of the deafblindness condition and if any type of assistive technology is used. The exclusion criteria include age below 18 years and do not being literate.
The participation in the experimental activity was voluntary and all individuals involved completed anamnesis questionnaire and filled in a free informed consent form, containing information about the procedures to be performed in the experiment, as well as the purpose for which this research is performed. The volunteers had the right to join or stop the tests at any time, according to their wish, without any penalty.
The sample size of the experiment consisted of 7 deafblind individuals. Among the volunteers, three are women and four are men. From those, 67 % of the feminine group have high education, where 100 % of the masculine group having only elementary school. The average age is 47.75± 4.19 years for men and 42± 5.57 years for women.
After that, Semmes-Weinstein Monofilaments, also called esthesiometers, were used to analyze the integrity of sensitivity in the extremities of the sample fingers. The monofilaments provide a non-invasive evaluation of cutaneous sensation levels throughout the body with results that are objective and repeatable [13]. Its use is indicated in diagnoses including nerve compression syndromes, peripheral neuropathy, thermal injuries, and postoperative nerve repair.
The Semmes-Weinstein Monofilaments [14] consists of a standardized set of nylon filaments, varying in length and diameter. These filaments are made in various and standardized colors according to the thickness, to provide a tactile scale of sensitivity perception.
The sensitivity test was performed as shown in Fig. 2, with the green filament, of the smallest thickness (0.05 g). If any sample member responds that they do not feel the sensitivity of this filament, the analysis proceeds to the blue filament, of greater thickness (0.2 g), and so on, for the violet (2.0 g), red (4.0 g), orange (10.0 g) and pink (300 g) filaments. Through this preliminary analysis, the exclusion criterion for participating in the study was defined as the report of sensitivity deficit for the green filament, that is, if there is a report of non-sensitivity to the green filament, the sample member is not able to participate in the survey.
Three prototypes were used for version 1, which will be called prototypes 1a, 1b and 1c, and three prototypes for version 2, which called prototypes 2a, 2b and 2c. The tests were conducted over six days, one day for each prototype, and consisted of the volunteer positioning his hands on the prototype. The data collection period between the tests with prototypes 1a, 1b and 1c and prototypes 2a, 2b and 2c was 6 months. This was the period needed to make a version 2 that contemplated all the suggestions given by the sample volunteers. After all, part of the product development process must go through the validation of potential users, especially in the case of deafblind persons, who have particularities often unknown by the project development team. The opinions of the deafblind users were of fundamental importance for the development of a product consistent with their needs.
Fig. 2Semmes-Weinstein monofilament evaluation. Source: Authors
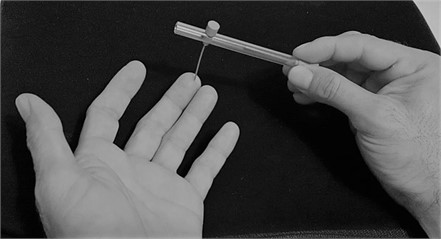
On each finger, successively, a triaxial accelerometer was fixed and the measurement of the emitted vibrations was collected during one minute for each finger, the minimum time required to collect reliable data for the analytical calculations of the vibrations. Fig. 3 shows the instrumentation used on one of the sample volunteers.
Fig. 3Equipment and instruments used in this research. Source: Authors
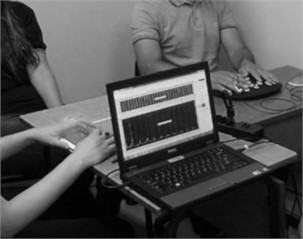
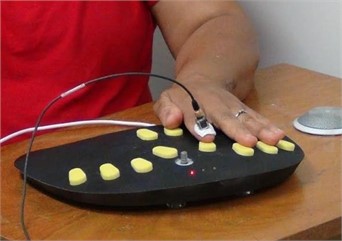
The equipment used were Sony omnidirectional 360-degree microphone model PCS-A1, Sony HDR XR260V HD camcorder, Samsung Galaxy Tab E SM-T561 8GB 3G 9.6" Android tablet, a handheld triaxial cubic accelerometer model Delta Tron 4524-B-001 from Brüel & Kjær manufacturer, a Phothon II dynamic signal analyzer from LDS-Dactron manufacturer and Dell Latitude E6430 I5 8gb 320gb HD Notebook with IBM SPSS Statistics software.
3. Results
All volunteers showed normal sensitivity in the evaluation region of the skin that corresponds to the pulp of the fingertips, in other words, all reported sensitivity to the green filament (0.05 g). The stimulus was performed in all fingers, but randomly, and the volunteer had to answer which finger was being sensitized with their eyes closed, since they had different levels of blindness.
To verify the vibration levels that users would be exposed, Eq. (1) was used to obtain the total vibration acceleration value measured in , and directions. With this value, Eq. (2) was used to find time to the Daily Vibration Exposure for each finger of the volunteer. The average of these results can be seen in Fig. 4 expressed by a boxplot graph in which each finger is represented on the -axis and is represented on the -axis. The order of finger numbering follows the sequence: number 1 is the pinky finger of the left hand, and so on until finger 5, which corresponds to the thumb of the left hand; finger 6 corresponds to the thumb of the right hand, and so on until finger 10, which corresponds to the pinky finger of the right hand.
Fig. 4The effective frequency-weighted acceleration value
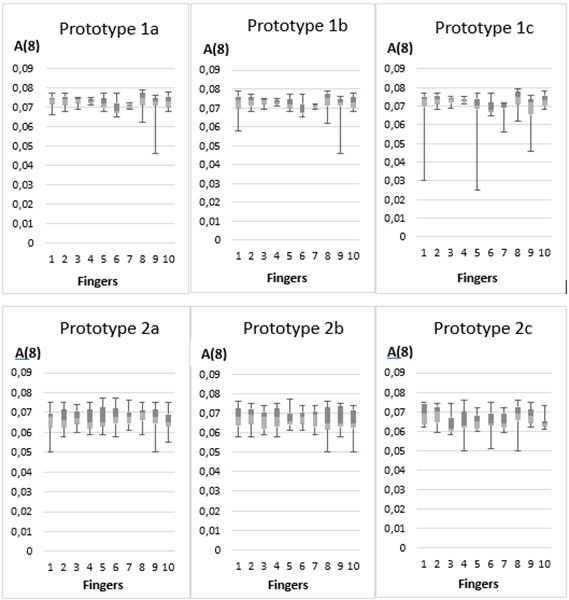
The Fig. 4 shows the results for each finger with the percentiles, mean, and median below . Note that the results of each finger using each device had the percentiles, mean and median, considering a time of 8 hours of exposure to hand and arm vibration, which would be the time equivalent to a daily workday, which would allow deafblind person to use the tested equipment for communication in some company and allow the inclusion of deafblind persons in the labor market, for example.
Fig. 5Exposure in years to acquire Raynaud’s syndrome
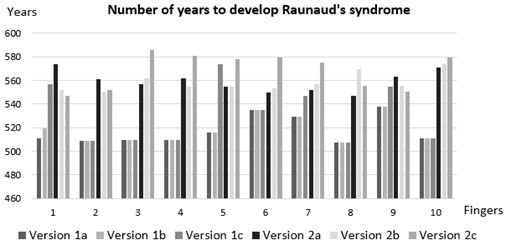
The expected estimate that 10 % of people exposed to hand and arm vibration may develop Raynaud’s syndrome in a given number of years was calculated using Eq. (3). The result for each finger of each volunteer using the 6 devices is shown in Fig. 5.
The device is safe for daily use, with 10 % of person only developing a disease over 500 years old, that is, about 7.5 times the world life expectancy, for both genders. In all situations, the data are within acceptable limits set by the standards and the likelihood of the onset of Raynaud’s syndrome was higher than the life expectancy reported in the World Health Statistics 2021 [15], where, based on the recent risks of mortality due to COVID-19 pandemic, men will live on average 70.9 years-old and women, 75.9 years-old.
4. Discussion
Vibration transmitted to the hand-arm system is the second largest problem of vibration on the human body, the first being exposure to whole-body vibration (WBV). While the vibration transmitted to the body standing or sitting increases problems of a general nature, such as discomfort, nausea and reduced work efficiency, the vibration applied to the hand can, in addition, produce localized physical damage due to high exposure levels. The vibration levels found in many vibrating hand tools and devices can be high enough to cause damage when operated for long periods of time, including musculoskeletal disorders [16].
The vibration can be transmitted to the body through one or two hands leaning against a vibrating device. For low levels of vibration there will be discomfort and reduced work efficiency. For high levels and long periods of exposure, diseases occur that affect blood vessels, joints and circulation [17].
The development of Raynaud’s syndrome is approximately five times more common in women than in men and this syndrome is so severe that in some cases individuals need to quit their job or profession and cannot perform activities that involve contact with vibration, either receptive or produced by the movement of touch [18]. The diagnosis of Raynaud’s syndrome is complex and should be made by a series of medical exams, analyzed by professionals in this area [19].
In a study using rats exposed to vibration, a progressive reduction in intact sensory nerve endings was observed in the tail tissue of rats upon increasing vibration exposure from 1 min to 12 min [20]. According to the literature, two types of experiments were proposed [21] to verify the effects of vibration on hands and arms in 16 men between 27 and 50 years old who had no experience with the operation of hand-operated vibration tools. The first experiment analyzed the effects of vibrations with a large amplitude of acceleration on the muscles of the hand and arm under conditions of room temperature and normal pressure. In his second experiment, it was sought to analyze the effects of vibration on muscles under conditions of high pressure and low ambient temperature, around 10 °C. In both experiments, there was a relationship between these variables and the impact caused by exposure to vibration.
Cumulative exposure to manual vibration considering the influence of vibration magnitude parameters, lifetime exposure duration, and vibration frequency can trigger the development of Raynaud’s syndrome [22]. It is important to maintain low vibration levels to prevent neurological damage to the hands, and it is suggested that there is a dose-response relationship between vibration exposure and numbness of the hands [23]. in a study from Sweden, it was identified that 21 % of the employees in a Swedish mechanical industry presented symptoms related to hand and arm vibration exposure, despite the relatively low exposure period. In addition, associated cases of carpal tunnel syndrome were identified [24].
In a study that aimed to evaluate the transmissibility of the elbow towards the forearm with constant grip force application at three levels (20 %, 30 % and 40 %), it was found that the increase in force, despite not changing the first resonant peak at 8 Hz, increased the second resonant frequency and the vibration transmissibility at higher frequencies [25]. This study demonstrates a relationship between force application and vibration. In Caeski, force is applied to press the buttons, but the vibration reception occurs when the muscles of the fingers are not in contraction, in this way, so there are chances of lower transmissibility.
Based on a literature review, published with a view to the effects of exposure to hand-arm vibration, or segmental vibration, the authors identified the presence of hand arm vibration syndrome in the subjects under heavy work, like rock drill and concrete breaker [26]. The exposure to hand and arm vibration as a function of concentration, intensity and exposure time from handheld devices may cause health damage if not within the safe exposure criteria outlined in ISO 5349-1: 2001 [10] and European Directive 2002/44/EC [9].
Motivated by the physical risk evidenced in specialized literature resulting from exposure to vibration in the upper limbs, the vibrations emitted by Caeski were analyzed and have acceptable levels in accordance with the main applicable international standards. Other assistive technologies also use vibrations, such as the Lorm Gloves [27] that have vibrating sensors on their surfaces and the Vibraille/Vibrotactile Alphabets [28] that consist of the vibration of Braille cells and, in both cases, an accurate analysis of the vibrations emitted by these devices should be encouraged.
5. Conclusions
Social inclusion is everyone’s right, and for that, engineering has a role in developing technologies that enable individuals with special needs to overcome daily challenges. The assistive technologies break down the barriers that the real world imposes on certain groups, mainly to get positions in the industry and on everyday situations. Nonetheless, when developing any type of technology, it is important to check all interfaces so that such a device, despite being the solution to a particular problem does not generate another and cause disruption to the user’s life.
From the reasons mentioned in this study, it is important to consider the harmfulness of a handheld device that produces vibration from its conception and to measure such vibration levels during the product development process so that substitutions and adaptations can be made, if necessary. The Caeski, a communication device developed for deafblind persons presented in this paper, can be safely employed regarding the vibration levels emitted by its motors. It may only cause some sort of discomfort, but that was not reported among the volunteers tested. Therefore, it’s may objective was achieved with success.
References
-
T. Choudhary, S. Kulkarni, and P. Reddy, “A Braille-based mobile communication and translation glove for deaf-blind people,” in 2015 International Conference on Pervasive Computing (ICPC), pp. 1–4, Jan. 2015, https://doi.org/10.1109/pervasive.2015.7087033
-
J. C. Cunha, R. V. Cendon, and P. Nohama, “Assistive technology for deafblind individuals,” Jor. Bras. Neuro, Vol. 20, pp. 53–72, 2009.
-
G. Llorach Tó, “Gesture recognition and interaction with a glove controller: an approach with a glove based on accelerometers,” Ph.D. dissertation, Univercity of Adelaide, Adelaide, Australia, 2013.
-
O. Ozioko, W. Navaraj, M. Hersh, and R. Dahiya, “Tacsac: a wearable haptic device with capacitive touch-sensing capability for tactile display,” Sensors, Vol. 20, No. 17, p. 4780, Aug. 2020, https://doi.org/10.3390/s20174780
-
E. E. Abdallah and E. Fayyoumi, “Assistive technology for deaf people based on Android platform,” Procedia Computer Science, Vol. 94, pp. 295–301, Jan. 2016, https://doi.org/10.1016/j.procs.2016.08.044
-
M. J. Griffin, Handbook of Human Vibration. London (United Kingdon): Academic Press Limited, 1996.
-
L. Goldman and A. I. Schafer, “Other peripheral arterial diseases,” in Goldman’s Cecil Medicine, New York, NY, USA: Elsevier Health Sciences, 2012, pp. 288–301.
-
R. Huebner and T. Ramos Luz, “Communication device for deafblinds: Testing with users for software and hardware enhancements,” in 24th ABCM International Congress of Mechanical Engineering, Jan. 2017, https://doi.org/10.26678/abcm.cobem2017.cob17-2102
-
“Minimum health and safety requirements regarding the exposure of workers to the risks arising from physical agents (Vibration),” European Directive 2002/44/EC, 2002.
-
“Mechanical vibration. Measurement and evaluation of human exposure to hand-transmitted vibration – Part1: General Requirements,” ISO 5349-1:2001, 2001.
-
“Human response to vibration – Measuring instrumentation – Part 1: General purpose vibration meters,” ISO 8041-1, 2017.
-
“Mechanical vibration. Measurement and evaluation of human exposure to hand-transmitted vibration – Part 2: Practical guidance for measurement at the workplace,” ISO 5349-2, 2001.
-
A. Ramachandran, L. Safar, S. Sasidharanpillai, K. Ajithkumar, B. George, and K. Devi, “Sensitivity and specificity of traditional testing methods to detect sensory impairment in the skin lesions of leprosy compared to Semmes-Weinstein monofilaments,” Leprosy Review, Vol. 91, No. 1, pp. 89–99, Mar. 2020, https://doi.org/10.47276/lr.91.1.89
-
S. C. Shen and R. A. House, “Hand-arm vibration syndrome: what family physicians should know,” Canadian Family Physician, Vol. 63, No. 3, pp. 206–210, 2017.
-
“World health statistics overview (2019) monitoring health for the sustainable development goals,” World Health Organization, 2020.
-
L. E. Charles, C. C. Ma, C. M. Burchfiel, and R. G. Dong, “Vibration and ergonomic exposures associated with musculoskeletal disorders of the shoulder and neck,” Safety and Health at Work, Vol. 9, No. 2, pp. 125–132, Jun. 2018, https://doi.org/10.1016/j.shaw.2017.10.003
-
Leoncio Ladeira de Mendonça Neto and Maria Lúcia Duarte, “Effects of vibration on human during cycling: hand arm vibration evaluation,” in 23rd ABCM International Congress of Mechanical Engineering, Jan. 2015, https://doi.org/10.20906/cps/cob-2015-0868
-
I. C. Chetter, P. J. Kent, and R. C. Kester, “The hand arm vibration syndrome: a review,” Cardiovascular Surgery, Vol. 6, No. 1, pp. 1–9, Dec. 1997, https://doi.org/10.1016/s0967-2109(97)00090-2
-
A. L. Herrick, “Raynaud’s phenomenon,” Journal of Scleroderma and Related Disorders, Vol. 4, No. 2, pp. 89–101, Feb. 2019, https://doi.org/10.1177/2397198319826467
-
J. Zimmerman, J. Bain, M. Persson, and D. Riley, “Effects of power tool vibration on peripheral nerve endings,” International Journal of Industrial Ergonomics, Vol. 62, pp. 42–47, Nov. 2017, https://doi.org/10.1016/j.ergon.2016.08.012
-
C. Heaver, K. S. Goonetilleke, H. Ferguson, and S. Shiralkar, “Hand-arm vibration syndrome: a common occupational hazard in industrialized countries,” Journal of Hand Surgery (European Volume), Vol. 36, No. 5, pp. 354–363, Feb. 2011, https://doi.org/10.1177/1753193410396636
-
R. House, M. Wills, G. Liss, S. Switzer-Mcintyre, L. Lander, and D. Jiang, “The effect of hand-arm vibration syndrome on quality of life,” Occupational Medicine, Vol. 64, No. 2, pp. 133–135, Mar. 2014, https://doi.org/10.1093/occmed/kqt167
-
M. Edlund et al., “Quantitatively measured tremor in hand-arm symptoms in a Swedish mechanical industry,” International Journal of Industrial Ergonomics, Vol. 62, pp. 77–81, 2017.
-
P. Vihlborg, I. L. Bryngelsson, B. Lindgren, L. G. Gunnarsson, and P. Graff, “Association between vibration exposure and hand-arm vibration vibration-exposed workers,” International Archives of Occupational and Environmental Health, Vol. 88, No. 3, pp. 305–310, 2015.
-
E. Marchetti et al., “An investigation on the vibration transmissibility of the human elbow subjected to hand-transmitted vibration,” International Journal of Industrial Ergonomics, Vol. 62, pp. 82–89, Nov. 2017, https://doi.org/10.1016/j.ergon.2017.04.001
-
S. V. Deshmukh and S. G. Patil, “Influence on physical work while working with segmental vibration inducing hand operated power tools,” International Journal of Engineering and Technology, Vol. 4, No. 5, pp. 308–323, 2012.
-
U. Gollner, T. Bieling, and G. Joost, “Mobile Lorm Glove: introducing a communication device for deaf-blind people,” in TEI’12: Sixth International Conference on Tangible, Embedded, and Embodied Interaction, pp. 127–130, Feb. 2012, https://doi.org/10.1145/2148131.2148159
-
X. Liu and M. Dohler, “Vibrotactile alphabets: time and frequency patterns to encode information,” IEEE Transactions on Haptics, Vol. 14, No. 1, pp. 161–173, Jan. 2021, https://doi.org/10.1109/toh.2020.3005093
About this article
This research was only possible through the partnership between the Bioengineering Laboratory (LabBio-UFMG) and Group of Acoustics and Vibrations in Human Beings (GRAVISH-UFMG) from Universidade Federal de Minas Gerais.
The datasets generated during and/or analyzed during the current study are available from the corresponding author on reasonable request.
Taciana R. Luz: conceptualization, investigation, methodology, project administration, validation, visualization, writing – original draft preparation, writing – review and editing. Rudolf Huebner: funding acquisition, project administration, resources, supervision. Maria Lúcia M. Duarte: data curation, formal analysis, methodology, resources, software. Herbert C. Nick: data curation, formal analysis, methodology, software, writing – original draft preparation.
The authors declare that they have no conflict of interest.
This research was approved by the Research Ethics Committee of the Universidade Federal de Minas Gerais through Certificate of Presentation for Ethical Appreciation (CAAE) number 09925612.9.0000.5149.