Abstract
The article's authors examined the possibilities of intensifying the lyophilization process in an innovative sublimation device with the help of ultrasonic vibration. The introduction of lyophilization technology on an industrial scale began relatively recently. Manufacturers widely used this technology to process high-value food products, primarily berries, vegetables, and fruits. The purpose of freeze drying is to reduce the products' mass and preserve the product’s properties for a long time. Lyophilization involves the removal of water from the frozen product, sublimation, and desorption under high vacuum conditions. The process aims to obtain the desired residual moisture value in the final product. However, the process implementation is associated with large consumption of the required energy. The high energy costs and long time needed for lyophilization make it an expensive process compared to other drying technologies, especially in the case of freeze-drying fishery products. Therefore, the authors propose introducing ultrasonic vibrations to the product with the help of an advanced pin to create fine ice crystals inside target bodies and speed up the sublimation process without significantly impacting aesthetic properties.
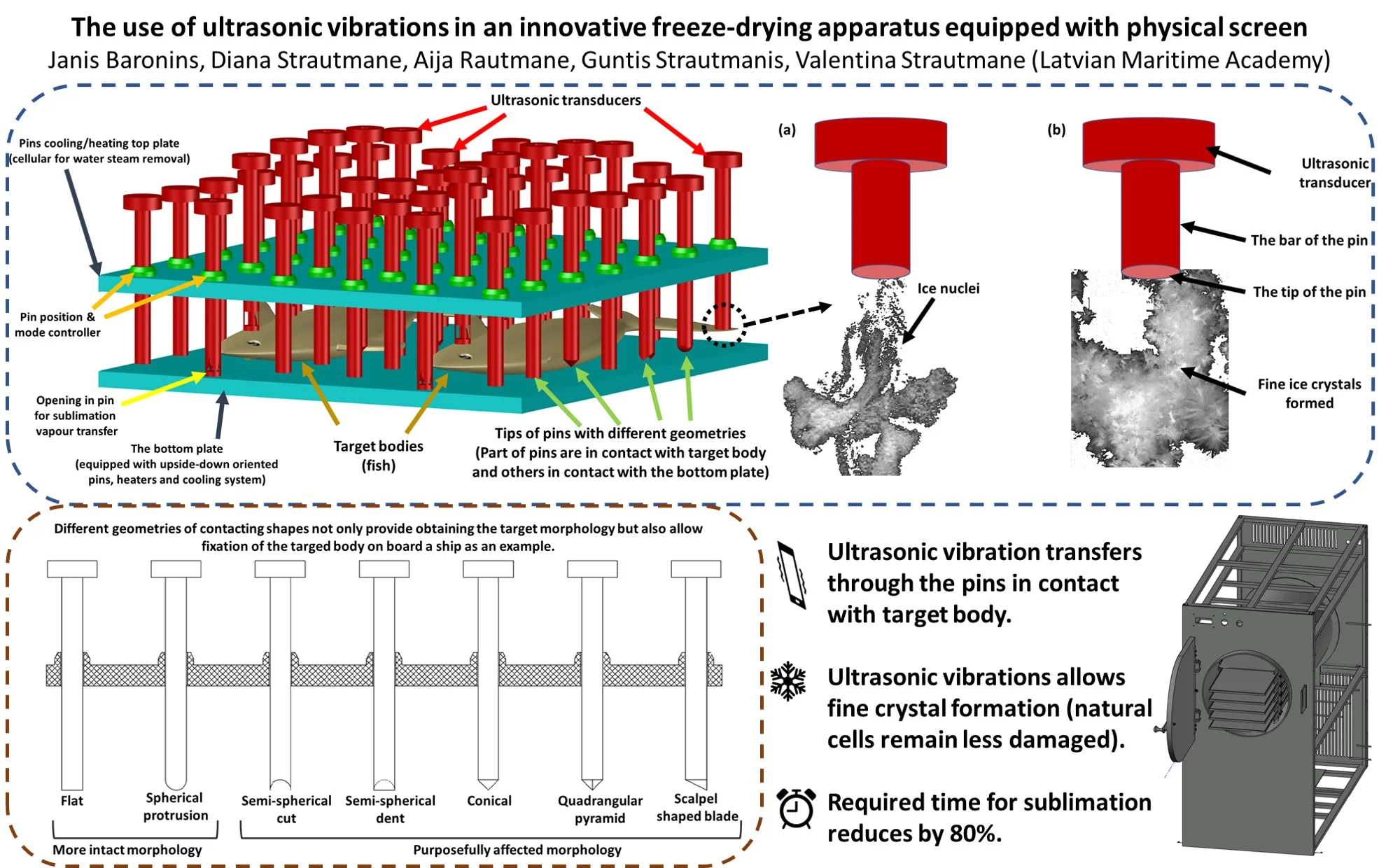
Highlights
- Ultrasonic vibrations transfers trough the pins in contact with target body.
- Ultrasonic vibrations allows fine crystal formation (natural cells remain less damaged).
- Required time for sublimation reduces by 80%.
1. Introduction
Dewatering is one of the most efficient approaches to ensure a long shelf life of foods. Cold and hot drying with or without simultaneous sterilization is the typical method for manufacturing canned food with a significant impact on the final product's structure, taste, and content. One of the possible production processes, which ensures a long shelf life of the product with preservation of flavour, design, and vitamins, is lyophilization (freeze-drying). This process removes the free water from the frozen product by sublimation and provides desorption of physically bounded water under high vacuum conditions.
The lyophilization process was justified and described for the first time in history in 1906 in their publication by Bordas and d’Arsonval, published by the Paris magazine Académie des Sciences [1] during the Second World War to ensure long-term storage of human plasma [2]. The freeze-drying process aims to obtain the desired residual moisture value in the final product. However, the implementation of the process is associated with large consumption of the required energy. The high energy costs and long time needed for lyophilization make it an expensive process compared to other drying technologies [3]. Therefore, the most significant efforts are focused on reducing the time required for drying while maintaining the planned product quality [4], [5].
Designing a successful lyophilization cycle requires determining the optimal value of shelf temperature, air (or gas), the vapor pressure in the working chamber, and process duration. In the three stages of the lyophilization process (freezing, primary drying, and secondary drying), Researchers in the literature distinguishes six main physical phenomena. These phenomena significantly impact the process's course, the quality of the obtained food product, as well as the total costs of the process. These phenomena are:
– transition of the liquid water phase in the food product into the ice phase;
– transition of the ice phase into the vapor phase (sublimation);
– desorption of water molecules from the structure of the food product;
– ensuring sufficiently low pressure (high degree of dilution) in the working chamber;
– re-sublimation of water vapor removed from the material on the surface of the condenser;
– removing the ice layer from the surface of the condenser itself.
The only feature of the lyophilization process that significantly distinguishes it from vacuum drying is the need to keep the unbound water frozen, which is one of the most challenging problems. Lyophilization is a mass transfer process that requires heat transport.
Too low heat energy input intensity slows down the lyophilization process and increases its cost. Conversely, too high heat flow causes an undesirable accumulation of heat energy in the structure of the target body to be freeze-dried. Undesirable formation of liquid water may lead to target body structural deformations and colour changes. Therefore, it is essential to balance the amount of heat supplied and consumed in the process. One of the ways to assess whether the amount of heat provided is too high is to monitor the temperature of the lyophilized material [6]. Its value must not exceed the cryoscopic (freezing point) temperature for a given material or the amorphous phase transition temperature for determined water volume inside the target body. Additionally, the exceed in the amorphous phase transition temperature may lead to a reduction in the porosity due to the compaction of the structure. This effect is very disadvantageous due to the decrease in the specific surface area of the target body for evaporation of sublimated water. As a result, the time of the second drying stage increases, the rehydration ability of the product deteriorates, and the resulting lyophilized product has a higher total water content [7], which can lead to the lower stability of the product during storage.
The freezing process generates many tiny ice crystals with a high mass transfer resistance inside the matrix of the target object to be freeze-dried. On the other hand, large ice crystals cause a relatively small resistance [8]. However, to intensify the secondary drying period, the ice crystal sizes should be smaller as possible. It helps to ensure the largest possible surface area to the matrix of the target body to be dried and water for evaporation from the matrix. However, such conditions cause more intensive desorption of unfrozen water from the pores on the surface of the amorphous matrix [8]. Therefore, an appropriate freezing method is possible after analysing the primary and secondary freeze-drying processes. If secondary drying takes a long time, the acceleration can be provided by adjusting the freezing conditions to obtain many tiny ice crystals.
Ensuring the formation of fine ice crystals and the theoretical possibility of increasing the speed of the lyophilization process with the help of ultrasound are discussed in this article.
2. Schematic of innovative lyophilization equipment
The authors propose an innovative freeze-dried drying equipment design, as shown in Fig. 1. A physical screen (pinboard) allows more efficient sublimation of seafood with complex shapes (such as fish, crustaceans, squid, etc.). Therefore, manufacturers can produce freeze-dried products more efficiently and environmentally friendly. Furthermore, the innovative device allows the production of high-quality and environmentally friendly products with a longer shelf life and more intact or customized cuts with the help of sharp tips in the target body's original structure (or morphology). Therefore, an essential aesthetic characteristic may improve consumer appetite. In addition, this technology allows more efficient preservation of valuable natural components (fatty acids, omega-3 substances, vitamins, etc.) [9-10].
Introducing a screen with highly thermally conductive pins in the sublimation chamber allows for more efficient heat removal. In addition, with the help of pins, it is possible to apply ultrasonic vibration during the freezing stage, which forms tiny ice crystals in the product [11], as demonstrated in Fig. 2.
Fig. 1Schematic of the device with a physical screen and an example of the use of movable pins
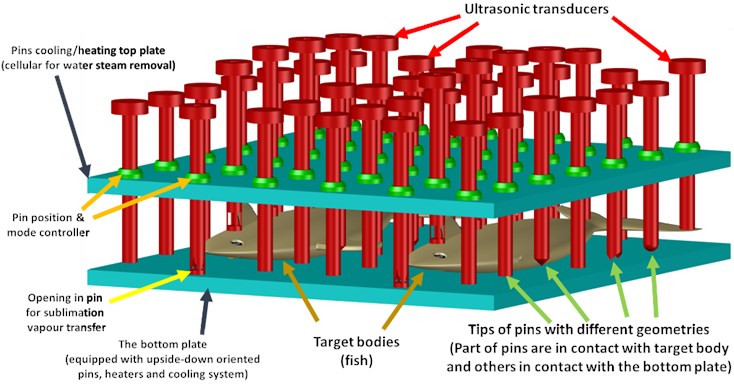
Fig. 2Schematic effect of ultrasonic vibrations on ice crystal sizes. Photographs of ice crystals nucleated in a 15 wt. % sucrose solution at −3, 4℃ by a commercial ultrasonic device (output 4, 10 % duty cycle)
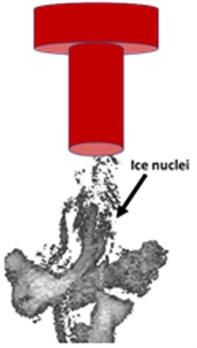
a) Ice crystals following an ultrasonic pulse
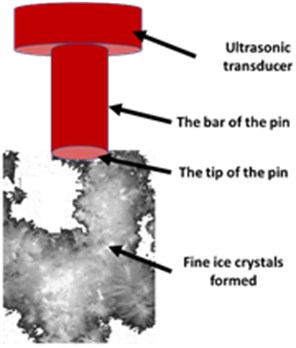
b) Crystals 5 s later
With the pins, it is also possible to ensure a more efficient heat energy supply for an increased steam formation rate in the sublimation stage. At the same time, ultrasonic vibration, which transfers to the target body with the help of pins, additionally intensifies the sublimation process [12]. As follows from the publication, low-intensity ultrasonic vibration makes it possible to reduce the sublimation drying time by 80 % [12], as demonstrated in Table 1.
Table 1Effective diffusivity (De), explained variance (VAR) and drying time (t) of air freeze drying experiments [12]
Power US (kW·m-3) | 0 | 10.3 | 20.5 | 30.8 |
De (m2·s-1) | 6.98·10-11 | 3.39·10-10 | 5.24·10-10 | 6.87·10-10 |
VAR (%) | 99.86 | 99.30 | 98.90 | 98.85 |
(h) | 80.0 ± 1 | 15.0 ± 0.3 | 10.0 ± 0.2 | 7.0 ± 0.1 |
So, the innovative lyophilization equipment, equipped with a physical screen and heated pins and through which ultrasonic vibration transfers to the product during the freezing and sublimation periods, ensures the formation of fine ice crystals and significantly reduces the time of the sublimation process.
3. Conclusions
A physical screen with heated pins and ultrasonic vibration, which transfers through the pins, allows to reduce the time of the sublimation process by 80 %, as well as improves the product quality with the formation of fine water ice crystals during the freezing of the fish product. The improvement of product quality is ensured by the fact that ultrasonic vibrations inhibit the formation of large ice crystals that can tear the cells in the fish, especially on the surface, which significantly affects the morphology and the related aesthetic properties.
References
-
Louis Rey, “Glimpses into the realm of freeze-drying: classical issues and new ventures,” Freeze-Drying/Lyophilization of Pharmaceutical and Biological Products, pp. 15–42, Apr. 2016, https://doi.org/10.3109/9781439825761-4
-
F. Franks, “Freeze-drying of bioproducts: putting principles into practice,” European Journal of Pharmaceutics and Biopharmaceutics, Vol. 45, No. 3, pp. 221–229, May 1998, https://doi.org/10.1016/s0939-6411(98)00004-6
-
B. Roser, “Trehalose, a new approach to premium dried foods,” Trends in Food Science and Technology, Vol. 2, pp. 166–169, Jan. 1991, https://doi.org/10.1016/0924-2244(91)90671-5
-
R. Pisano, D. Fissore, S. A. Velardi, and A. A. Barresi, “In-line optimization and control of an industrial freeze-drying process for pharmaceuticals,” Journal of Pharmaceutical Sciences, Vol. 99, No. 11, pp. 4691–4709, Nov. 2010, https://doi.org/10.1002/jps.22166
-
H. Sadikoglu and A. I. Liapis, “Mathematical modelling of the primary and secondary drying stages of bulk solution freeze-drying in trays: parameter estimation and model discrimination by comparison of theoretical results with experimental data,” Drying Technology, Vol. 15, No. 3-4, pp. 791–810, Jan. 1997, https://doi.org/10.1080/07373939708917262
-
D. Nowak, “The innovative measurement system of the kinetic of freeze-drying and sorption properties of dried products as a tool for controlling and assessing the course of freeze-drying,” 2017.
-
S. M. Patel, T. Doen, and M. J. Pikal, “Determination of end point of primary drying in freeze-drying process control,” AAPS PharmSciTech, Vol. 11, No. 1, pp. 73–84, Mar. 2010, https://doi.org/10.1208/s12249-009-9362-7
-
G. Assegehegn, E. Brito-De La Fuente, J. M. Franco, and C. Gallegos, “The importance of understanding the freezing step and its impact on freeze-drying process performance,” Journal of Pharmaceutical Sciences, Vol. 108, No. 4, pp. 1378–1395, Apr. 2019, https://doi.org/10.1016/j.xphs.2018.11.039
-
L. Kathleen Mahan and S. Escott-Stump, Krause’s Food and Nutrition Therapy. Philadelphia: Elsevier - Health Sciences Division, 2008.
-
F. A. F. de Castro et al., “Fatty acid composition of three freshwater fishes under different storage and cooking processes,” Food Chemistry, Vol. 103, No. 4, pp. 1080–1090, Jan. 2007, https://doi.org/10.1016/j.foodchem.2006.10.002
-
X. Ma, J. Mei, and J. Xie, “Mechanism of ultrasound assisted nucleation during freezing and its application in food freezing process,” International Journal of Food Properties, Vol. 24, No. 1, pp. 68–88, Jan. 2021, https://doi.org/10.1080/10942912.2020.1858862
-
C. Brines, A. Mulet, J. V. García-Pérez, E. Riera, and J. A. Cárcel, “Influence of the ultrasonic power applied on freeze drying kinetics,” Physics Procedia, Vol. 70, pp. 850–853, 2015, https://doi.org/10.1016/j.phpro.2015.08.174