Abstract
Direct-action and indirect-action vibrators for compaction of silage in container stores were built for experimental trials. Experimental results indicated that during vibrating compaction the grass layer was compacted intensely for 5-10 min. Therefore, vibrators of this type are suggested for compacting grass layers with thicknesses of 0.5-0.6 m. The determination of comparative pressure as a function of vibration frequency showed that the most effective frequency was 43.96 Hz during the direct-action vibrator compaction of grass. This relationship was determined experimentally by measuring the pressure caused by the vibrator and the effective power while compacting the grass mass in a container. The pressure increase coefficient varied over a wide range of 1.5-6.8. It was established that a centrifugal indirect-action vibrator works well to compact chopped maize (a bulk density of up to 730 kg m-3 was achieved) and works to some extent for mixtures of Caucasian goat’s rue (Galegaorientalis Lam.) and red clover (Trifoliumpratense L.). This vibrator is not suitable for compacting either pure red clover or pure Caucasian goat’s rue because the compacted densities were only 260-350 and 225-280 kg/m3, respectively. Similar studies were performed using a direct-action vibrator, and similar fodder compaction results were obtained. These vibratory silage compaction methods can be recommended for small farms. Vibrating devices are useful on herbaceous plants with large stems (e.g., maize) and are somewhat useful for mixtures including such herbaceous plants when compacting in stores.
1. Introduction
The predominant fodder for feeding cattle worldwide is silage, and more and more herbaceous plants are used for silage preparation in European states. Ensiling grass-based forage has been and will continue to be the primary way to preserve these plants as fodder. This study relates specifically to Lithuania, where the production of chopped grass silage in trenches, clamps and bales is predicted to remain constant in the near future. On small farms, silage container stores are sometimes used, and the silage is preserved in large (4-6 m3) boxes [1, 2].
In traditional silage plants, certain dietary components (e.g., proteins, fats) are sometimes missing or are present in low concentrations. As a result, these traditional fodders are overused. Such dietary gaps could be filled by growing non-traditional plants such as Goat’s rue (Galegaorientalis Lam.), which has the same nutritive value as lucerne. These crops can also be grown in hilly areas, sink-soils and other ecologically sensitive territories. Moreover, they grow perfectly in soils without nitrogen fertilisers, so they are suitable for ecological, or organic, farming [3, 4].
In addition to the traditional means of silage preparation, silage is prepared in both container and sectional storage [5]. These technologies are used in small and average sized farms. In such stores, appropriately chopped grass (with lengths of 20-30 mm forming 80-90 % of the mass) is stored. The grass is gradually placed in layers 0.3-0.5 m thick and compacted using different simple systems, e.g., by hand and by using additional weights. Vibratory compactors are used now only for research purposes, and we are proposing them as a new technology for small farms.
Theoretical and experimental trials were performed by evaluating factors such as the thickness of the grass mass, the influence of moisture content on the density and quality of the silage and the pressure of the silage mass on the vertical walls of the trench [6, 7]. All trials used traditional grasses and legumes such as clovers, timothy grass and lucerne. This study analysed the performance of silage-making technology in container stores using non-traditional protein fodder crops. In particular, this study focuses on Goat’s rue, which grows well in Lithuania, and mixtures of herbaceous plants (e.g., maize) that contain substantial amounts of sugar and are easy to silage.
In Lithuania, silage is usually prepared in trenches or clamps. Lately, the production of non-chopped silage in rolls and packs has also become more popular. Small farms may use containers for making silage, and silage production in containers does not require substantial capital. In Lithuanian, the Institute of Agricultural Engineering at Aleksandras Stulginskis University has designed and proposed sectional-container silage storage for small farms where fodder is grown for 3-5 animals. This store holds approximately 3 tonnes of silage with a density of approximately 600 kg/m3 and a moisture content of 60-65 %.
When using vibrating compactors, it is important to establish the frequency at which the fodder mass is best compacted, the mass of the equipment and the area that it can compact. Compacting with different wheeled tractors initially resulted in mass deformation of approximately 280 mm. The results showed that during compaction with tractors, the vertical force increased at certain frequencies of the tractor engine’s revolutions. In the latter stages of crop compaction, deformation decreased and the specific resonance frequency of the compacted crop mass increased [8].
A literature review showed that vibration methods of grass mass compacting in container stores had not been analysed thoroughly and instead had only received theoretical analysis [1, 5, 9]. Some years ago, investigations of vibrating compaction using vibrating rollers were performed, but the studies used a vibrating method suitable only for compacting in trenches and clamps rather than containers [10, 11].
To use equations for forced vibration, such a system can be analysed as a concentrated mass on a spring with one end tightly fixed and with internal friction present. The elements of mass resilience and mechanical resistance corresponding to its internal friction are separated from each other. We choose to influence the system by using a sinusoidal excitation force [12, 13].
Vibrating compactors consist of the compaction surface (either a plate or a roller), the vibrator and a gear. Compactors are classified into plate and roller types and are further classified into inertial, eccentric, hydraulic, electromagnetic or pneumatic [9]. Inertial vibrators are most suitable for silage compaction.
The power that an inertial vibratorevokes is produced by rotating weights. The evoked power, , equals [14]:
where is the power evoked by vibrator (N), is the mass (kg), is the radius of the weight spinning (m) and is the angular velocity of the weight spinning (s-1).
The gradual movement around the circle is periodic, and after some time, the movement repeats. During one such period of time, , the radius subtends an angle of rad. Therefore, the angular frequency (in this case, the vibrating angular velocity) equals:
where is the vibrating time per revolution (s) and is the number of revolutions during the period of time (s-1).
Vibrators with only one weight are an indirect-action type. Direct-action vibrators have two weights rotating in different directions at the same angular frequency. Such vibrators are more complex and expensive than indirect-action vibrators. Direct-action vibrators are suitable for silage compaction, and it is useful to study their efficacy and efficiency and to compare their operation parameters with indirect-action vibrators, whose construction is simple and they are less expensive [9, 14].
Vibrating compactors of various constructions for compacting fodder in trenches and container stores are found in Russia, Hungary, Germany and elsewhere. An electric, trailing vibrating compactor installed in a cylinder is generally used in trench-type clamps. The main disadvantage of such compaction is that the tractor, needed to tow the compactor, also compact the mass with its wheels. Moreover, the tractor can pollute the silage. Thus, self-propelling compactors are used for the container and sectional stores because they do not require tractors. Such compactors can be inert with one or two changeable weights, and these compactors can be either direct or indirect [12, 15, 16].
Using a vibratory method, the required input needed to compact the grass is similar to that when using tractors. However, with vibrating compaction, the tractor can be used for transportation, and silage can be produced in nonstandard or sectional types of trench. Additionally, oil products do not pollute the fodder, and there is less risk of injury because the operator is distant from the process. Therefore, for using a vibratory method to silage the mass, it is advisable to evaluate the mass compacting process, to select and evaluate compactors for chopped grass compaction, to determine the parameters of the compactors’ operation and to evaluate the parameters’ influences on the grass compacting process [9].
The aims of the research presented here were to evaluate the process and the technology of silage compaction via a vibrating method, to determine density and pressure changes for plants of different physical properties when compacted by direct-action or indirect-action vibrators, to determine the working characteristics of these vibrators and to evaluate the resulting silage quality.
2. Experimental methods
The research objectives included study of different forage plants: chopped maize (experiment 1), a mixture of maize and Caucasian goat’s rue (Galegaorientalis Lam.) (experiment 2) and Caucasian goat’s rue (experiment 3) compacted by a direct-action vibrator. A mixture of maize and red clover (experiment 4) compacted by an indirect-action vibrator was also studied. The plants had a moisture content of 68-85 % and were chopped with a Maral-125 forage harvester (chop length 15-20 mm). Trials were performed at the research laboratory of Institute of Agricultural Engineering, Lithuanian University of Agriculture (IAE LUA), and laboratory rigs were used for research experiments (Fig. 1 and Fig. 2).
Grassy plants and their mixtures were compacted in a storage container (measurements of a container: width, 0.75 m; length, 1.20 m; height, 0.95 m) with a 0.25×0.25 m pressure-sensitive plate at the bottom.
Research experiments and analysis of information sources were carried out in order to prove the optimality of the vibrators construction and main parameters. Taking into account the parameters chosen for the measurement of the container and compacted herbaceous plants,carried out on the basis of previous research, results have been calculated, and the optimal constructive parameters of vibrational equipment were selected: optimal weight of the vibrator plate (with the engine), optimal weight of the disbalance, optimal frequency of vibrations, etc.
For fodder compaction, two experimental inertial directional vibrators were used, both produced at the IAE LUA:
1) The centrifugal indirect-action vibrator was powered by an alternating current asynchronous engine of 1.1 kW power and 2850 min-1 revolution frequency. The size of the plate in contact with the forage was of 0.60×0.90 m, and the mass of the weights on the vibrator was 5 kg. The total mass of the centrifugal indirect-action vibrator was 113 kg.
2) The centrifugal direct-action vibrator consisted of two masses rotating in opposing directions. The centrifugal direct-action vibrator was powered by an asynchronous engine of 1.1 kW operated at 1500 min-1. Weights of 7.0 kg were fitted to rollers in the vibrator with their centres of mass located 40 mm from the axis. These mechanisms were fitted on a 0.60×0.90 m plate, and the total mass of this vibrator was 123 kg.
Fig. 1Scheme of grass compaction in container storage using centrifugal indirect-action vibratory device: (1) container storage (width, 0.75 m; length, 1.20 m; height, 0.95 m); (2) centrifugal indirect-action vibrator; (3) electrical engine; (4) changeable weights; (5) vibration sensor; (6) mass of compacted grass; (7) electric current frequency converter; (8) tensometer sensor; (9) pressure measurement device; (10) vibration measurement device
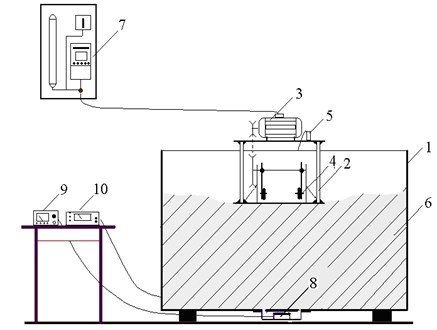
Fig. 2Scheme of grass compaction in container storage using centrifugal direct-action vibratory device: (1) container storage (width, 0.75 m; length, 1.20 m; height, 0.95 m); (2) centrifugal direct-action vibrator; (3) electrical engine; (4) weights; (5) vibration sensor; (6) vibrator cover; (7) electric current frequency converter; (8) tensometer sensor; (9) pressure measurement device; (10) vibration measurement device; (11) mass of compacted plants
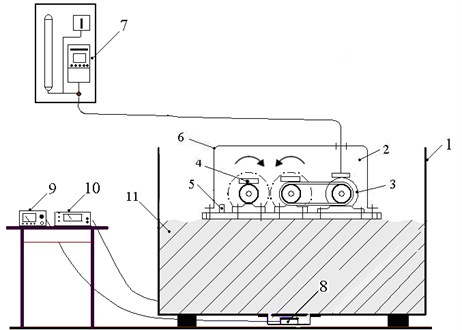
At the beginning of a trial, the container was loaded with chopped plants, and the vibrator was fitted on top and vibrated in one position for 20-30 min. The vibrator compacted approximately the whole surface of the container, with only approximately 0.12-0.15 m remaining between the compacted area and the walls of container. The plates that contacted the forage were changeable and could be exchanged for plates of different sizes to accommodate containers of different dimensions. Every 5 minutes during operation, the height of the fodder layer, , was measured. The heights were measured only while the vibrator was on the forage. After removing of the vibrator from an area, the forage rebounded to some extent and reduced the density. The rebound was not measured.
During the trials, two separate experimental variations were performed with the following main compaction parameters:
1) For determining the density variation of the plants and their mixtures as a function compaction duration, a layer of compacted grass 0.5-0.6 m and two layers were loaded into the container;
2) For determining the influence of vibration frequency and grass layer thickness upon pressure, a compacted grass layer of 0.45-0.95 m and 1-3 layers were loaded, and vibrator frequencies of 21.96 Hz, 43.96 Hz and 65.94 Hz were used.
For accurate evaluation of the influence that the vibrator’s excitation force has on the pressure of compacted grass, the pressure was recalculated as the relative pressure, , which represents the percentage increase of pressure in a grass layer during vibration relative to the pressure when only the vibrator’s gravity force is applied [9]:
where is the relative pressure (%), is the pressure in the grass layer while applying only the vibrator’s gravity force (kPa) and is the pressure in the grass layer during vibrator operation (kPa).
For determining a vibrator’s operational efficiency, we calculated the contact pressure created under the flat surface:
where is the pressure caused by vibrator (kPa), is the vibrator’s excitation force (N), is the vibrator’s gravity (N), is the pressed surface area (m2) and the pressure increase coefficient.
Eq. (4) shows that the pressure created, , depends on the total effect of the vibrator’s excitation, the gravity forces and the dimensionless pressure increase coefficient The coefficient’s value depends mainly on the ratio of excitation and gravity forces and was established experimentally.
Fig. 3Scheme of tensometer sensor: l – sensitive plate; 2 – body of tensometer; 3 – liner of tensometer; 4 – membrane of tensometer; 5 – tenso-converter; 6 – fastening screw of sensitive plate; 7 – body of tenso-block
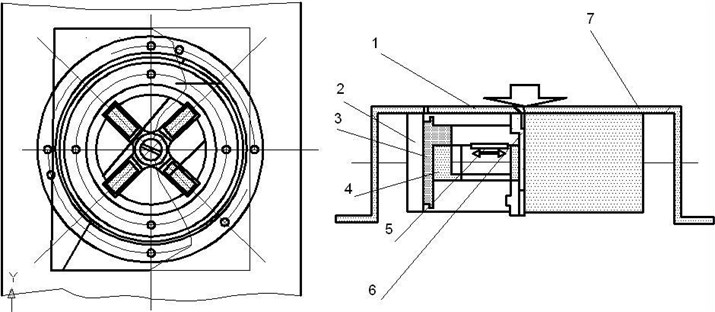
The pressure created by the static and dynamic forces on the surface of the plant mass and at the bottom of the container, where the tensometer sensor was fitted (Fig. 3). The pressure on the tensometer sensor was measured by a pressure measuring device, model 1526, from a Danish company, “Bruel&Kjaer”. For determination of the vibratory system’s resonance characteristics, vibration accelerations and velocities on the vibration plane of the direct-action vibrator were measured. Three experimental levels of vibration within the 1-27 Hz range were measured. Velocity and acceleration of the direct-action vibrator’s vibrations were measured according to the widely used methodology using a vibrometer, model 2511, with a band-adjustable filter (model 1621) manufactured by the same Danish company [9, 14].
The commutation scheme of pressure (tension) measuring and the mechanical and electrical schemes of tensometer sensor DST 0.8-0.6-2 No 5199 had been redesigned in order to increase measuring sensitivity and to adjust it to pressure measuring equipment. The sensitive element of the tensometer sensor was membrane 4 of the dimensional design. Tenso-converters 5 were stuck on its wings on both sides, and they transformed the pressure transmitted from the sensitive plate into an electrical signal. During the vibrator’s operation, grass mass pressure was transmitted to the tensometer fitted at the bottom of the container. Its electrical signals were registered by tensoindicator 1526. The complete system of the measuring equipment consisted of three main blocks: tensoindicator 1526, channel switching, commutation block 1544 and control and digital information block 1545.
All pressure measuring equipment was calibrated; schedules of meter calibration had been made. The tensometer was calibrated after taking it out of the tenso-block body and fitting it on a specially manufactured calibration stand.
The vibrators’ operation parameters, variation of grass mass density and other parameters were recorded using the methods described in the above. Using this system, the dependence of density on compaction duration was determined for maize, Caucasian goat’s rue, red clover and their mixtures for both inertial direct-action and indirect-action vibrators. The grass mass compaction efficiency was assessed according to variation of mass density, (kg/m3). The dry matter (DM) amount in compacted plants and the density of dry matter were also calculated. The volume of plant mass was calculated using a ruler (accuracy ±0,001 m) for measuring at the four corners of the container. The layers of plant mass were weighed using scales with a range of 10-100 kg (accuracy of ±0.1 kg).
After loading two 60 kg layers into the container and the subsequent compaction of both layers, the fodder was sealed by polyethylene film and pressed with a 100 kg mass load. Two months later, after storage in similar ambient weather conditions, silage samples were taken, and the quality of feed was evaluated using standard methods. To determine silage quality, the samples (3, each weighing 1 kg) were selected from three silage containers, which were filled with the chopped maize stems, Caucasian goat’s rue and mixture of maize-Caucasian goat’s rue. The pH, amount of dry matter, amount of ammonia, amount of green protein (total nitrogen content of the protein and non-protein nitrogenous substances) and digestibility of organic matter (part of the organic matter digested by animals, measured by the “in vitro” method) were measured using standard methods. The amounts and ratios of lactic, acetic and butyric acids were also determined [1].
All experiments were replicated three times. Each experiment was performed with one batch of forage harvested in one day but treated in separate containers. For the forages that were stored for two months, there were three containers for each crop. The research data were processed using mathematical-statistical methods by calculating the arithmetic mean and the average square deviation and by selecting Student’s coefficient (errors at 0.95 reliability).
3. Experimental results and discussion
Measurements during the compaction of red clover by an indirect-action vibrator showed that, depending on the vibrator’s work parameters and the physical characteristics of the grass and layer thickness, the pressure during the experimental period varied from 9.6 to 19.2 kPa. The basic characteristics of compacted red clover were as follows: dry matter content of 79.0 % and a maturity to flowering stage. By applying Eq. (3), the pressure was recalculated as comparative pressure, , and the data are presented in Fig. 4.
Fig. 4The influence of vibration frequency and grass layer thickness upon pressure during compaction of red clover by an indirect-action vibrator: 1 – vibrator rotation frequency ω= 21.96 s-1, grass layer thickness h= 0.95 m; 2 – ω= 43.96 s-1, h= 0.95 m; 3 – ω= 65.94 s-1, h= 0.95 m; 4 – ω= 43.96 s-1, h= 2×0.61 m; 5 – ω= 43.96 s-1, h= 3×0.45 m
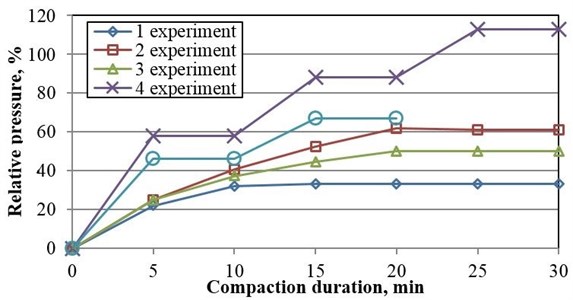
The first three curves in Fig. 4 demonstrate the influence of vibration frequency on the increase of comparative pressure when the layer thickness is constant. A relative pressure ranging from 33 % to 61 % is achieved, but the greatest relative pressure is achieved when vibrating at a frequency of 43.96 s-1 (curve 2). When the vibration frequency decreases or increases (curves 1 and 3), the comparative relative pressure decreases. Based on these results, compaction of red clover or other plants with similar physical-mechanical properties is recommended at a vibration frequency near 43.96 s-1 while using an indirect-action vibrator.
Two other curves in Fig. 4 (curves 4 and 5) represent data obtained when the vibrator operates at a constant frequency of 43.96 s-1 while changing only the thickness of the fodder plant layer. Jumps in these curves represent loading of new layers. Until the 5th minute of vibration, comparable relative pressures increase and then stabilise by the 10th minute. After 10 minutes, a new layer of fodder is loaded, the vibrator is switched on, and compaction continues until the 15th minute, when the relative pressure is constant. Based on these data, it is advisable to compact fodder layers of 0.5-0.6 m thickness, and fodder compaction is expected to compresses intensively for 5-10 min.
To prove the assumptions that resonance characteristics of the system depend on spring resilience and that amplitude characteristics depend on the mechanical resistance coefficient (i.e., internal friction of plant mass), the vibration accelerations and velocities on the vibration plane of the direct-action vibrator were measured [14]. The results of three experimental levels of vibration within the 1-27 Hz range are shown in Fig. 5.
The resonance frequency of the measured fodder compaction system varies depending on the fodder crop’s physical-mechanical properties. The masses of different plant types are characterised by different resilience characteristics. When compacting goat’s rue and its mixtures with other plants of higher resilience, vibration amplitude increases. The results showed that that a high acceleration is one of the most important settings for providing the highest density in a reasonable amount of time. Frequencies of 15 Hz for maize and 7 Hz for Caucasian goat’s rue produced the greatest accelerations.
The calculated and experimentally established pressure and effective force indicators of plant mass compaction while using the direct and indirect-action vibrators are given in Table 1. This table presents only the final results of the calculations and does not show the intermediate data. The experimentally established pressure increase coefficient varied widely and ranged from 1.5-6.8.
Fig. 5Characteristics of amplitude frequency while compacting chopped grass forage using a direct-action vibrator: (experiment I) mass of chopped maize; (experiment II) mixture of maize and Caucasian goat’s rue; (experiment III) chopped Caucasian goat’s rue
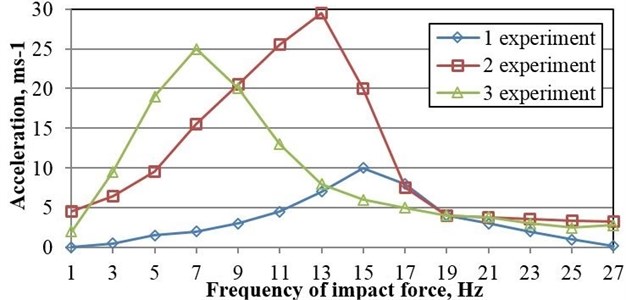
Table 1 presents the experimental and calculated results and shows that while using the direct-action vibrator, efficient mass pressing does not depend on the pressure increase coefficient. This coefficient depends on a chosen vibratory effective force frequency and mechanical resistance. This case proves the assumption that while using the vibrator, horizontal and vertical excitation force components appear, and as the vibrator frequency and effective force amplitude increase, the vibrator’s operation becomes unstable and the compacting efficiency decreases.
Our calculations of plant mass pressure show that vertical normal tensions were evaluated in the mass depth (at the container bottom) when the vibratory method was used for fodder plant compacting (Table 1). Better compaction is achieved when the pressing efficiency indicator is lower. By comparing the results of direct- and indirect-action vibrator experiments, we conclude that in some cases, an indirect-action vibrator compacts better than a direct-action one. We can also state that greater pressure force suppression leads to better compacting of the grass layer (the value of pressure force suppression repeatability is inversely proportional to the pressing efficiency). Additionally, when other conditions are similar, better-compacted fodder is characterised by greater mechanical resistance to the vibrator’s excitation force.
Table 1Comparison of vibrator pressure and force indicators
Mass compacting method (vibrator) | Calculated vibration pressure , kPa | Experimentally established pressure increase coefficient | Static pressure in grass mass (container bottom), kPa | Measured pressure increase due to vibratory effect, kPa | Pressure efficiency indicator (Pressure force suppression repeatability) |
Direct-action (exp. var. I) | 26.7 | 1.49±0.06 | 6.28±0.16 | 8.34±0.26 | 3.05 |
Direct-action (exp. var. II) | 26.7 | 6.82±0.18 | 10.22±0.30 | 13.83±0.43 | 5.07 |
Direct-action (exp. var. III) | 26.7 | 5.45±0.16 | 9.81±0.24 | 12.26±0.38 | 4.50 |
Indirect-action (exp. var. IV) | 37.3 | 3.35±0.12 | 3.92±0.14 | 9.81±0.19 | 3.33 |
The efficiency of silage compaction by centrifugal direct-action and indirect-action vibrators was evaluated during experimental trials. It was established that the fodder layer compacts intensively for 5-10 min and that further compacting is unnecessary, as there was no significant compacting or comparative pressure increase. Therefore, vibrators of this type are suggested for compacting fodder layers of 0.5-0.6 m thickness.
To fully explore the use of a vibratory device for silage production capabilities, the research was conducted with maize, Caucasian goat’s rue, red clover, maize-Caucasian goat’s rue mixture and maize-red clover mixture. Table 2 presents data showing the influence of compaction duration on the density for these plants when the forage is compacted by a centrifugal indirect-action vibrator that was powered by an alternating current asynchronous engine of 1.1 kW and 2850 min-1 revolution frequency. For these experiments, the plant moisture content was 68-74 %, and the material was chopped with the Maral-125 forage harvester (chop length 15-20 mm).
The influence of compaction duration on variation of red clover density is presented in Table 2. The analysed vibrator did not achieve a high density of red clover mass. After 20 min of compacting a 120 kg layer, the achieved density was 315 kg/m3 and consisted of only 88 kg/m3 dry matter (DM). After 10 min of compacting a 60 kg layer, the achieved density was 350 kg/m3 with less than 100 kg/m3 dry matter. The density was not sufficient to produce high quality silage, and this vibrator was not suitable for compacting red clover.
When compacting Caucasian goat’s rue, the density was higher – up to 147 kg/m3 DM. However, this density is also not sufficient for high quality silage production.
Table 2 shows the influence of compaction duration on maize mass density when the fodder is compacted by a centrifugal indirect-action vibrator. The moisture content of the maize mass was 67.3±0.4 %. This trial achieved a rather high density of maize mass. After 20 min of compacting 120 kg maize mass, the achieved density was 730 kg/m3 with 239 kg/m3 of dry matter. After compaction of the first maize mass layer (60 kg) for 10 min, 808 kg/m3 (264 kg/m3 of dry matter) density was achieved. Densities of 600 kg/m3 [1] are sufficient to produce high quality silage.
Table 2The influence of compaction duration on variation of herbaceous plant density
Compaction duration , min | Herbaceous plant density , kg/m3 | ||||
Red clover | Caucasian goat’s rue | Maize | Maize and red clover mixture (mixture rate 2:1) | Maize and Caucasian goat’s rue mixture (mixture rate 2:1) | |
10 | 350 | 280 | 808 | 620 | 528 |
100 (DM) | 147 (DM) | 264 (DM) | 192 (DM) | 155 (DM) | |
20 | 315 | 225 | 730 | 530 | 425 |
88 (DM) | 118 (DM) | 239 (DM) | 178 (DM) | 124 (DM) |
The vibrating compactor was efficient when used on the maize and red clover mixture. The mixture density was similar to that of maize. After 20 min of compaction (2 layers of 0.60 m), a 530 kg/m3 density was achieved. Compacting the first 60 kg layer even resulted in 620 kg/m3 density after 10 min. Dry matter density was also sufficiently high (192 kg/m3 and 178 kg/m3, respectively) for the first and second layers (Table 2).
Research results of compaction duration influence on the density of a 2:1 mixture of maize and Caucasian goat’s rue showed that investigated the centrifugal indirect-action vibrator did not achieve a high density of the mixture. After 20 min of compacting 120 kg of the mixture, the density was 425 kg/m3. After 10 min, the density of a 60 kg mass was 528 kg/m3. The dry matter densities were 124 kg/m3 and 155 kg/m3, respectively (Table 2). These densities are not sufficient for silage.
Based on these experimental data, we can state that the indirect-action vibrator design would be useful for compacting maize (reaching a density of 730 kg/m3 with 239 kg/m3 of dry matter) and that the design is partly satisfactory for compacting the maize-red clover and maize-Caucasian goat’s rue mixtures. This compactor is not suitable for compacting red clover or Caucasian goat’s rue.
Similar studies were performed using a direct-action vibrator, and the results showed similar forage compaction efficiency to that of the indirect-action vibrator. These results also support the proposition that vibratory compactors are suitable for compacting herbaceous plants with large stems (e.g., maize) and are somewhat suitable for compacting mixtures with such plants.
While assessing the technology of grass compaction in a container storage by using vibratory thickening, the main focus was paid to grass mass chopping, loading into the container and compaction by using the vibratory mechanism (harvesting and transporting operations were not analysed). This technology was assessed by three parameters: time required to perform each operation and the complete production cycle, production efficiency and the quality of feed.
All grass silage production in the container stores during vibratory devices cycle is equal to the sum of the following technological operations (min):
– duration of grass chopping;
– duration of grass loading into container;
– duration of lifting up vibrator;
– duration of grass compaction;
– duration of sealing and pressing grass in container.
Technological indicators of grass compaction are presented in Table 3. Depending on consistency and parallelism of technological operations, 25-50 min are needed to perform a production cycle with a full container (150 kg of grass). At adequate planning and distribution of operations, a silage production cycle (without grass harvesting and transporting operations) can be shortened down to 10-25 min, i.e., down to the total time of carrying out vibrator lifting up and down and grass compaction operations.
The efficiency of a production cycle was limited by a technological operation of the lowest efficiency. In this case, it was grass thickening – 0.5-0.8 t/h-1 (Table 3).
Table 3Technological indicators of vibratory grass thickening
Technological indicators | Technological operations | |||||
Grass chopping | Grass loading into container | Vibrator’s lifting up and down | Grass compaction | Grass sealing and pressing | Complete production cycle | |
Duration of technological operation , min | 8-10 | 3-5 | 4-5 | 5-20 | 5-10 | 25-50 |
Efficiency of technological operation , t h-1 | 1-1.5 | 2-3 | 0.5-0.8 | – | – | |
Note: in the line of the table “Duration of technological operation” (line two), the time was registered when the operation was carried out with 150 kg of grass. |
Silage quality was determined from three silage containers filled with chopped maize, Caucasian goat’s rue and the maize-Caucasian goat’s rue mixture. The determined silage quality indicators are presented in Table 4.
Table 4Results of silage quality analysis
Silage | pH contents | Dry matter, % | Green protein, % | Ammonia, % | Digestibility, % |
Maize | 3.9 | 26.3 | 8.5 | 1.3 | 85 |
Caucasian goat’s rue | – | 29.5 | 15.8 | 2.4 | 65 |
Maize and Caucasian goat’s rue mixture | 4.3 | 32.5 | 11.8 | 1.8 | 87 |
Feed quality analysis (DM amount, pH, amount of ammonia and green protein, digestibility of organic matter and amount of acids) established that maize silage met the requirements for the highest class silage, that a maize-Caucasian goat’s rue mixture met the requirements for second-class silage and that the quality of pure Caucasian goat’s rue silage was very poor (the forage overheated, and there was a substantial amount of mould bacteria). The analysis results showed that in the maize and maize-Caucasian goat’s rue mixture silages, ammonia and lactic and acetic acids were at the correct levels, while the pH and butyric acid slightly exceeded acceptable levels. The measured pH values indicate good forage fermentation in both cases. The pH and fermentation products for Caucasian goat’s rue were not determined. The density of silage in the container stores varied from 550 till 620 kg/m3.
Based on these results a vibratory silage compaction method can be recommended for small farms. Vibrating compactors are useful for compacting herbaceous plants with thick stems (e.g., maize) and are somewhat useful for mixtures of such plants when compacting in container stores.
4. Conclusions
The efficiency of silage compaction by centrifugal direct-action and indirect-action vibrators was evaluated via experimental trials. It was determined that the forage layer compacts intensively for 5-10 min. Further compaction time is inappropriate as there was no significant compaction or comparative pressure increase. Therefore, it is suggested that vibrators of this type can be used for compacting layers of 0.5-0.6 m thickness.
The results of compaction with indirect-action vibrators, along with the dependence of comparative pressure on vibration frequency, show that the most effective vibration occurs at a frequency of 43.96 Hz.
The resonance frequency of the measured silage compaction system varies depending on the fodder mass’s physical-mechanical properties. Masses of different plant origins are characterised by different resiliencies. When compacting Caucasian goat’s rue and its mixtures with other plants of higher resilience, the vibration amplitude increases.
Calculated and experimentally established pressure and effective force indicators were determined for grass compaction in container stores while using the direct and indirect-action vibrators. The experimentally established pressure increase coefficient varied over a wide range, from 1.5 to 6.8.
It was shown that the centrifugal indirect-action vibrator is reasonable to use for compacting chopped maize (a bulk density up to 730 kg/m-3 was achieved) and is somewhat useful for mixtures containing maize. While compacting a maize-red clover mixture (layers of 0.60 m mass thickness), a satisfactory density of 530-610 kg/m-3 was achieved.
Similar studies were performed using a direct-action vibrator and obtained similar forage compaction efficiency results. A vibratory silage compaction method can be recommended for small farms. Vibrating devices are useful for plants with large stems (e.g., maize) and are somewhat useful for mixtures of such plants when compacted in container stores.
References
-
Sirvydis J., Dravininkas A. Artificially Dried Grass Forage Technology Basics: Monograph. Raudondvaris, 2005, p. 230.
-
Jasinskas A. The drying dynamics of red clover mats. Biosystems Engineering, Vol. 93, Issue 3, 2006, p. 261-268.
-
Spruogis V. Technologies of Goat’s Rue Growing for Seed and Feed. Kaunas, Akademija, 1999, p. 72, (in Lithuanian).
-
Baležentienė L., Sprogis V., Juknevičius S. Research of Goats rue (Galegaorientalis Lam.) nutritive value. LUA Research Papers, Vol. 59, Issue 12, 2013, p. 23-28.
-
Antonov N. M. The Technology of Vegetative Materials Thickening. Moscow, 1995, p. 55-65, (in Russian).
-
Darby D. E., Jofriet J. C. Density of silage in horizontal silos. Canadian Agricultural Engineering, Vol. 35, Issue 4, 1998, p. 72-75.
-
Langlen R. W. Agricultural structures: wall leads. Landwards, Vol. 55, Issue 4, 2000, p. 10-12.
-
Spirgys A. Safe Use of Low Power Tractors in Chopped Grass Packing. Ph.D. Dissertation, Lithuanian University of Agriculture, Kaunas, Akademija, 2001, p. 141.
-
Ferevičius D., Jasinskas A. Research of silage thickening of grasses mixtures using the vibrator of undirected action. Agricultural Engineering: IAE LUA and LUA Research Papers, Vol. 37, Issue 4, 2005, p. 16-27.
-
Häbler J., Tölle R., Hahn J. Vibratory rollers for compacting ensiling material. Landtechnik, Vol. 63, Issue 1, 2008, p. 28-29.
-
Hahn J., Tölle R. Precision Storage – ein Beitrag zur Informationslogistik. 16. Arbeitswissenschaftliches Kolloquium des VDI-MEG Arbeitskreises Arbeitswissenschaften im Landbau. Potsdam, Bornimer Agrartechnische Berichte Heft, 2009, p. 66, (in German).
-
Hegedűr A., Cizmadia B. Vibration analysis of two-mass vibratory screen. International Conference on Mechanical Vibration, Timiscara, 2002, p. 23-24.
-
Mikhlin Y. U., Vakakis A. F., Salenger G. Direct and inverse problems encountered in vibroimpact oscillations of a discrete systems. Journal of Sound and Vibration, Vol. 216, Issue 2, 1998, p. 227-250.
-
Augustaitis V. K. Basics of Mechanical Oscillatory Motion. Vilnius, 2008, p. 318.
-
Cizmadia B., Hegedűr A. Optimization the flexible elements of two-mass vibratory screen. Research papers. Hungarian Institute of Agricultural Engineering, Vol. 15, 2002, p. 44-46.
-
Babitsky V. I. Theory of Vibroimpact Systems and Applications. Springer, Berlin, 1998, p. 154.
About this article
The role of each co-author to the paper preparation. Algirdas Jasinskas was the research supervisor of this work, he prepared article methodical part, has organized and carried out the experiments with inertia vibrators (undirect and directed action), investigated the silo compaction process in a container storage, formulated the conclusions. Egidijus Šarauskis performed part of Information sources review, analyzed vibratory compaction equipment, prepared part of experimental tests methodology, managed research data in the graphs and conducted statistical analysis of these results and formulation of conclusions. Rolandas Domeika studied the undirect action vibratory compaction equipment, based vibratory compaction unit process operations and conducted the parameters of these technological operations, managed research data and conducted the conclusions formulation. Gintas Viselga analyzed the sources of information, presented theoretical assumptions of vibrational compaction equipment working process, prepared vibratory compaction equipment and labor survey methodology involved in their experimental studines. Vytautas Kučinskas investigated direct action vibratory compaction equipment, performed their experimental studies, analyzed technological operations of vibratory compaction unit working process and conducted of prepared feeds qualitative analysis. Edvardas Vaiciukevičius participated in the vibrational compaction equipment experimental studies, analyzed working process and technological operations of compactors, conducted by the analysis of statistical data and qualitative assessment of prepared charts.