Abstract
Mechanical impact generation systems available on the market today suffer from a lack of control of the generated shocks. In this paper authors present new design of the system for mechanical impact generation. Theoretical and experimental investigations are presented and they prove ability to generate mechanical impact and provide such metrological parameters, as amplitude of impact energy, acceleration, coefficient of restitution and velocity of impact. Experimental results showed that developed system generates impact and provides parameters, which characterizes the researched objects. Developed system for mechanical impact generation is an efficient tool for well defined mechanical impact generation in such areas as diagnostic systems of various materials, impact energy harvesting, testing building structures, etc.
1. Introduction
Nowadays mechanical impact is involved in a lot of technical processes, e.g. in the field of nondestructive evaluation of concrete [1], for structure borne sound analysis, for diagnostic system of composite materials [2], for impact energy harvesting [3, 4], for shockwave generation [5], etc. For all processes, mentioned above, well defined mechanical impact is necessary. For this purpose, a wide variety of impact generators are developed. Generally, it is two basic types of impact generators: mechanical and non-mechanical. The first ones can be operated by machine (e.g. pneumatic, hydraulic) or by human. This type of impact generator in common is based on the hammer-anvil principle [6], that is why this allow only a limited control of shock amplitude and pulse width with a limited repeatability of the applied impacts (e.g. depending on how the operator releases the hammer pendulum the shock amplitude can change up to 10 % and more). The second ones are usually based on piezoelectric principle, where piezoelectric actuator is used as generator [5]. Both types of impact generator create not only impact, but also provide parameters of impact such as amplitude, acceleration of impact, frequency of generated shockwave, etc.
The aim of this research is to design and investigate a new type of impact generation system, which could generate impact and provide such metrological parameters: amplitude of impact energy, acceleration, generated shockwave frequency, coefficient of restitution and velocity of impact.
2. Modelling of mechanical impact
To investigate mechanical impact parameters dependency from different colliding bodies material properties, the mechanical impact 2D axial symmetry computational model was created using ANSYS Explicit dynamics software. During this modelling impacting body was simulated as a sphere, made of steel 1006. This sphere has initial velocity and impacts into plate, which is fixed at the bottom and made of various materials – steel 1006 and aluminum AL6061-T6.
The computational model with boundary conditions and gauge points, consists of 420 finite elements with 481 nodal points, is presented in Fig. 1.
The modelling was carried out in two cases: the first modelling was carried out with sphere, made of Steel 1006 and plate, made of the same material. The second modelling was carried out with sphere, made of Steel 1006 and plate, made of the aluminum AL6061-T6. Table 1 shows geometric parameters of computational model, which was used in both calculations. Materials properties, used in modelling are presented in Table 2.
Fig. 1Computational model of mechanical impact: 1 – gauge point, located on the edge of the impact plate, 2 – gauge point, located on the edge of the impacting sphere
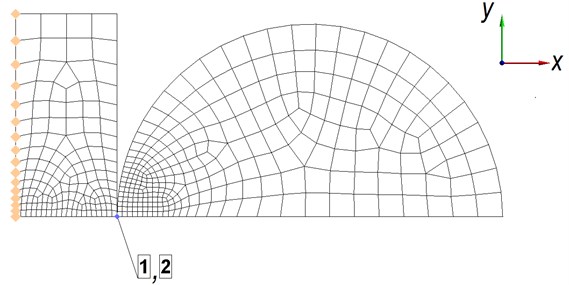
Table 1Geometric parameters of computational model
Parameter | Measurement unit | Value |
Radius of impacting sphere | mm | 19 |
Length x width impact plate | mm | 20×10 |
Initial velocity of impacting sphere | m/s | –1.1 |
Initial distance between impacting sphere and impact plate | mm | 0.05 |
Table 2Material properties, used in modelling
Parameter | Measurement unit | Steel 1006 | Aluminum AL6061-T6 |
Density | kg/m3 | 7896 | 2703 |
Gruneisen coefficient | – | 2.17 | 1.97 |
Shear modulus | GPa | 81.8 | 27.6 |
Yield stress | MPa | 350 | 290 |
The modelling results – directional ( direction, see Fig. 1) displacement of gauge point 1, which is located on the edge of the impact plate (see Fig. 1), are given in Fig. 2.
Fig. 2Modelling results – directional displacement of gauge point 1: a) the first modelling, sphere made of steel 1006, plate made of steel 1006, b) the second modelling, sphere made of steel 1006, plate made of aluminum AL6061-T6
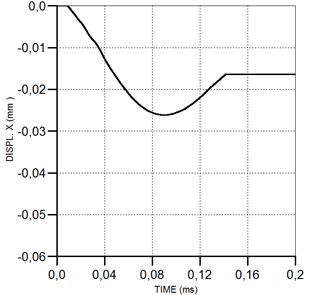
a)
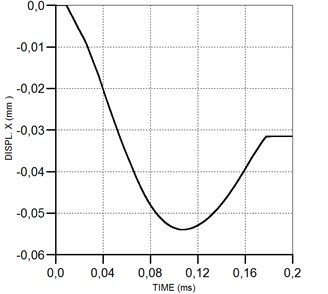
b)
The modelling results – directional ( direction, see Fig. 1) velocity of gauge point 2, which is located on the edge of the impacting sphere (see Fig. 1), are given in Fig. 3.
Modelling results showed, that maximum displacement of impact plate when colliding pares are made form steel 1006 and steel 1006 is 0,025 mm, the maximum displacement of impact plate when colliding pares are made form steel 1006 and aluminum AL6061-T6 is 0.055 mm. Directional velocity change time from –1.2 mm/s to –0.8 m/s is shorter when impacting sphere impacts into plate, made of steel (0.13 ms) than plate, made of aluminum (0.17 ms).
Fig. 3Modelling results – directional velocity of gauge point 2: a) the first modelling, sphere made of steel 1006, plate made of steel 1006, b) the second modelling, sphere made of steel 1006, plate made of aluminum AL6061-T6
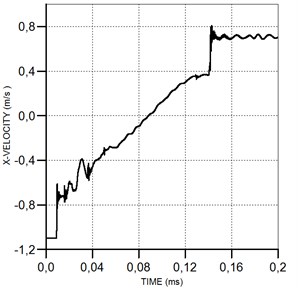
a)
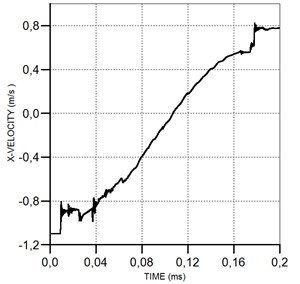
b)
3. Experimental investigations
In order to investigate dynamic parameters of mechanical impact, experimental research were carried out. Experimental research was carried out on the basis of the theoretical basis. Principal scheme of experimental setup is shown in Fig. 4.
Fig. 4Scheme of experimental setup: 1 – impacting sphere, 2 – accelerometer, 3 – potentiometer, 4 – impact plate, 5 – digital oscilloscope, 6 – power supply
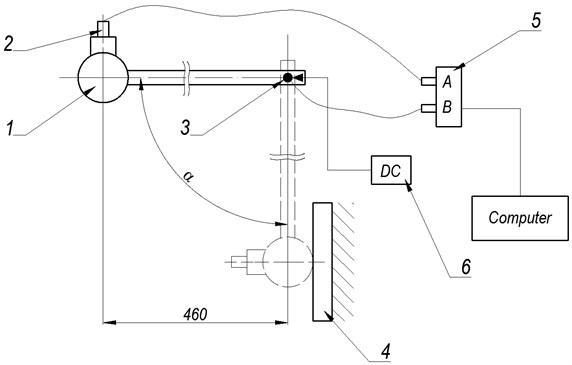
During experimental investigation impacting sphere 1 (see Fig. 4.) was released from certain height and as pendulum impacted into impact plate 4. For all experiments pendulum initial angle was 34°. Acceleration of impacting sphere was measured by accelerometer 2. Velocity of impact was measured by potentiometer 3. Data acquisition from the sensors and analysis was performed with PC. For the analysis of the experimental results MATLAB software package was used.
Three types of experiment were made. First experiments were made when impacting sphere, made of hardened steel C45E impacted into plate, made of steel C45E, the next when same impacting sphere impacted into plate, made of steel AISI304 and the last one when impacting sphere (made of hardened steel C45E) impacted into plate, made of aluminum AL6061-T6. Experimental setup is shown in Fig. 5.
Fig. 5Experimental investigation setup: 1 – impacting sphere, which mass is 295 g, 2 – accelerometer KD91, 3 – potentiometer, 4 – impact plate, 5 – digital oscilloscope PicoScope 3424, 6 – power supply Mastech HY1803D, 7 – PC
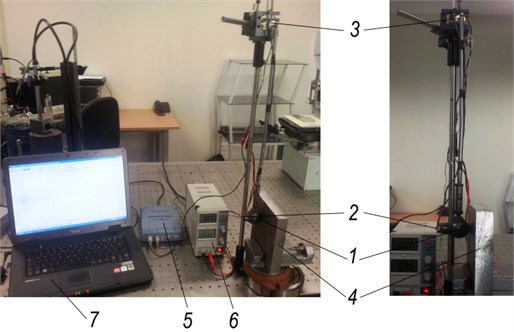
Fig. 6Experimental results – a) potentiometer voltage change during experimental investigation, b) accelerometer signal, impact plate made of steel C45E, c) accelerometer signal, impact plate made of steel AISI304, d) accelerometer signal, impact plate made of aluminum AL6061-T6
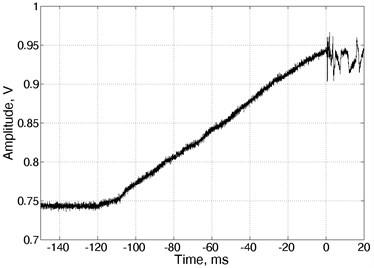
a)
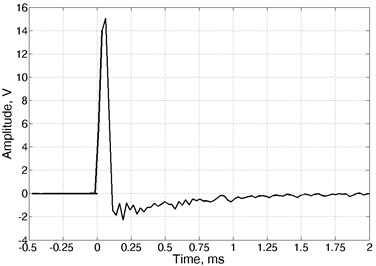
b)
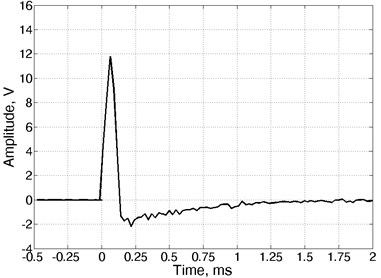
c)
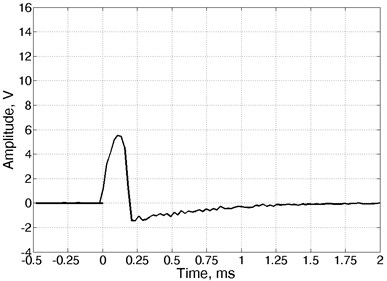
d)
In experimental results –voltage rate of potentiometer is proportional to velocity of impacting sphere. These results are presented in Fig. 6(a). Regarding to these results, potentiometer, which is mounted in pendulum axis (see Fig. 5), voltage rate during mechanical impact is known, thus velocity of mechanical impact was calculated ~1 m/s. Due to the fact, that impacting sphere material and releasing height has not changed during all experiments, it can be considered that velocity and initial conditions of mechanical impact were the same during all types of experimental investigations.
Experimental results – accelerometer signals, when conditions of mechanical impact are the same, are given in Fig. 6(b), (c), (d).
4. Conclusions
A new type of impact generation system has successfully been designed, researched and developed. In this paper presented system generates mechanical impact and by analyzing obtained modelling and experimental results could provide such mechanical impact parameters: amplitude, velocity, acceleration, force, impact time, coefficient of restitution.
Modelling of mechanical impact showed, that maximum displacement of impact plate when colliding pares are made form Steel 1006 and steel 1006 is 0.025 mm, the maximum displacement of impact plate when colliding pares are made form Steel 1006 and aluminum AL6061-T6 is 0.055 mm. Experimental research results showed that impact velocity when impacting sphere is released from height when pendulum initial angle 34° is ~1 m/s. The longest impact time is when impacting sphere impacts into plate, made of aluminum – 0.235 ms and the shortest when made of steel – 0.105 ms. The highest impact amplitude – 15 V was obtained when impacting sphere impacted into plate, made of steel C45E, the lowest – made of aluminum AL 6061-T9 – 5.8 V.
In this paper researched and developed system for mechanical impact generation has such technical characteristics: impacting sphere mass 295 g, diameter 38 mm, the maximum measuring acceleration 25∙103 m/s2, frequency range 1-104 Hz. In order to analyze generated shockwave, additional sensor could be used in the future.
Developed system for mechanical impact generation prove ability to characterize the colliding objects and could be used in such areas as diagnostic systems of various materials, impact energy harvesting, testing building structures, etc.
References
-
Tong J. H., Wu T. T., Lee C. K. Fabrication of a piezoelectric impact hammer and its application to the in-situ nondestructive evaluation of concrete. Japanese Journal of Applied Physics, Vol. 41, 2002, p. 6595-6600.
-
Zhou L., He Z., Sun H. Lamb wave mode conversion-based crack detection for plate-like structures without baseline information. Journal of Vibroengineering, Vol. 15, Issue 2, 2013, p. 647-657.
-
Jacquelin E., Adhikari S., Friswell M. I. A piezoelectric device for impact energy harvesting. Smart Materials and Structures, Vol. 20, 2011.
-
Sheng W., Hong H., Siyuan H. Modeling and experimental investigation of an impact-driven piezoelectric energy harvester from human motion. Smart Materials and Structures, Vol. 22, 2013.
-
Schad Hp., Pickelmann L. High power piezoelectric axial shockwave generation. Piezomechanik, Munich, 2008.
-
Nicklich H., Brucke M., Mende M. The need for controlled shocks – a new type of shock exciter allows to apply well defined mechanical shocks. XIXX IMEKO World Congress, Fundamental and Applied Metrology, Lisbon, Portugal, 2009.