Abstract
This study was conducted to suggest the potential use of a mechanical vibration stimulus in the ankle to correct gait abnormalities. As for the mechanical vibration stimulus, different locations and durations are suggested based on the detection results of real-time gait patterns. 5 young males participated in this study. They were asked to perform assigned gait tasks when either a threshold or sub-threshold stimulus was applied in the tibialis anterior and Achilles tendon. The analysis results of gait cycle and muscle activity showed the changes on gait cycle, the activity pattern of used muscle for gait and the movement pattern of the ankle were observed based on the applied locations of vibration stimulus. Also, the result of sub-threshold stimulus showed similar effects as that of threshold stimulus. As such, the mechanical vibration stimulus was considered to affect gait by being adjusted its characteristics and local stimulus also would affect human body systemically. The result of this study can be used as basic data for the correction of individual’s specific gait abnormality and rehabilitation using vibration stimulus.
1. Introduction
Gait occurs by the repetitive motion of the lower extremities, and can be summarized as the repetition of stride by setting the foot as a reference. Gait has a series of repeated cycles, and gait events are present in each cycle. Thus, a basic gait pattern can be assessed using changes in the gait event and cycle [1]. Gait is an activity that involves complex mechanisms that occur in various parts of the lower extremities, trunk, head, and arms. Normal gait requires a close cooperation of various motility systems including the musculoskeletal system [2]. However, it is difficult to conduct normal gait if the dysfunction of the motility systems occurs due to causes such as disease or aging.
Various studies on the complementation/improvement of the dysfunction of the motility systems have been in progress in order to overcome abnormal gait that may occur due to functional changes or dysfunction of the motility system. Gait disturbance, which occurs due to the dysfunction of the nervous, skeletal, and muscular systems that play an essential role in gait activity, is corrected via localized unicompartmental arthroplasty [3], functional electrical stimulation (FES) [4], and muscle strengthening [5], among which gait correction via FES and muscle strengthening has been reported to be effective in the treatment of gait disorders that occur due to the weakened muscle caused by aging in various studies [6]. However, FES acting on the muscle may cause problems such as the injury of the skin to which electrodes are attached, patient’s discomfort or pain upon electrical stimulus, and muscle fatigue caused by prolonged use.
To prevent these problems, efforts that recover the function of the muscular system using exogenously applied vibration stimulus have attracted attention. Humphries et al. [7] reported that vibration stimulus applied to athletes induced an increase in muscle strength and muscle activity. Galica et al. [8] reported that vibration stimulus applied to the soles of the feet of the elderly reduced the risk of falling by reducing gait variability. These previous studies aimed to demonstrate the usefulness of vibration stimulus through a variety of human responses that are changed by the application of vibration stimulus. However, few studies have been conducted to determine the characteristics of vibration stimulus, such as the application site, timing, and overall time of vibration stimulus in gait that occurs by the complex response of the musculoskeletal system. The gait pattern may vary depending on the interaction of agonist and antagonist muscles, and vibration stimulus plays a secondary role in the control of muscle activity by inducing muscle contraction. Thus, the characteristics and pattern of vibration stimulus should be investigated to utilize vibration stimulus for gait correction.
Accordingly, in this study, gait pattern was detected in real time using a footswitch mounted on the soles of the feet in order to increase the effectiveness of vibration stimulus. Based on the detected gait pattern, somatosensory stimulus that determines the application site and time of vibration stimulus was implemented. In addition, the changes in gait pattern that occur by the application of vibration stimulus were analyzed via the changes in the gait cycle and muscle activity. The results of this study can be used as a reference for determining the usefulness of vibration stimulus on gait correction, and can also be used as basic data for the correction and rehabilitation of individual-specific gait patterns using vibration stimulus.
2. Study methods
2.1. Equipments
The experiment was carried out in a motion analysis laboratory equipped with a camera for motion analysis and a force platform. Four force platforms (Bertec Co., USA) were constructed under a 10 m footpath, and three infrared motion analysis cameras (Optotrak Certus, Northern Digital Inc., Canada) were installed around the footpath. The force platform collected ground reaction forces that arise in the course of gait, and the motion analysis camera collected three-dimensional information of 15 infrared lightening diode markers attached to the lower extremities of the subjects according to the Helen-Hayes marker set. The motion analysis camera and force platform were synchronized to analyze marker data and ground reaction force data by time and interval.
The somatosensory stimulus system that applied vibration stimulus to the subjects was produced using the linear vibration device that generates mechanical vibration. The somatosensory stimulus system was designed to adjust the intensity of somatosensory stimulus that is applied to the body by controlling the electrical characteristics of the input power that drives the vibration device. In this study, stimulus with a vibration frequency of 250 Hz, which has the highest absolute sensitivity regardless of the contact area, was used [9]. The vibration stimulus of 250 Hz accepted vibration from the Pacinian corpuscle receptor and was then transmitted to the central nervous system using the PC (Pacinian corpuscle-associated) fiber. As the tibialis anterior tendon and Achilles tendon to which vibration stimulus is applied have a relatively high density of the Pacinian corpuscle receptor, the optimum efficiency was expected to be achieved. In addition, to change the application site, timing, and time of vibration stimulus according to subject’s gait cycle, a footswitch was used at the bottom of the first metatarsal bone and the calcaneus. Vibration stimulus was applied to the tibialis anterior tendon and Achilles tendon that play a major role in maintaining the balance of body posture.
2.2. Subjects and experimental methods
The experiment was conducted on 5 young males who had no musculoskeletal and neurological disease and who had normal gait pattern. Prior to the experiment, the purpose and procedures of the experiment were adequately explained to the subjects, and subjects voluntary consent on experiment participation was acquired.
Considering that the intensity of sensory stimulus recognized by each subject varies, the vibration stimulus threshold of each subject was measured for the tibialis anterior tendon and Achilles tendon before the initiation of the experiment. The sensory stimulus with an intensity of threshold, which was obtained via the aforementioned process, was set as threshold stimulus (TS) and the sensory stimulus with an intensity of 80 % of the threshold [10] was set as sub-threshold stimulus (STS).
After attaching the infrared-emitting markers and the somatosensory stimulator to subject’s lower extremities and ankle, respectively, the subjects were asked to walk straight on the 10 m footpath according to the instruction. The gait was performed at a speed preferred by the subjects, and vibration stimulus was randomly applied to increase the reliability of the measurements and to minimize sense compliance.
Fig. 1Experiment environment for gait analysis by applying somatosensory stimulus
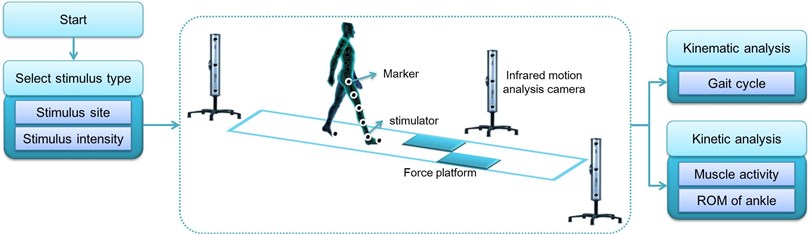
2.3. Data analysis
Gait events were set in order to quantify the movement of the subjects during their gait. The gait events were set based on the vertical ground reaction force among force platform data, and then classified into Loading Response (LR), Mid Stance (MSt), Terminal Stance (TSt), and Pre-A Swing (PS). Initial Contact (IC) was set as a point where ground reaction force is more than 10 N and Toe off (TO) was set as a point where ground reaction force is less than 5 N [11].
A kinematic analysis of gait was conducted using the percentage of the gait events. The stance phase was normalized considering the individual variation in gait time. Subsequently, the percentage of each gait event in the stance phase was calculated by comparing the time of the stance phase by stride with the time of the gait event.
The kinetic analysis of gait was conducted via gait simulation using a body model. The gait simulation model was constructed using three-dimensional coordinate data of the markers obtained using a motion analysis camera. Then, the muscle activity of the tibialis anterior (TA), extensor digitorum longus (ED), soleus (SO), and gastrocnemius (GC) that play a major role in gait was obtained via the body model. Body model construction, gait simulation, and the corresponding kinetic calculation were obtained using SIMM (Software for Interactive Musculoskeletal Modeling, MusculoGraphics Inc., USA).
A statistical analysis of all the data was conducted using SPSS 12.0 (Statistical Package for the Social Sciences, SPSS Inc., USA). The general characteristics of the subjects were analyzed using the mean and standard deviation. A paired t-test was conducted to test the significance of the experimental conditions within each group.
3. Results
This study analyzed the changes in the gait cycle and muscle activity that occurred when vibration stimulus whose characteristics were changed through the real-time detection of the gait event using a footswitch was applied to the body, and the following results were obtained.
3.1. Comparison of gait cycle
The percentage of the gait cycle according to the characteristics of vibration stimulus is presented in Fig. 2. The percentage of the gait cycle was obtained by calculating the time of each gait event based on the time of the entire stance phase of stride.
When the threshold stimulus was applied to the tibialis anterior, the percentage of the loading response, mid stance, terminal stance, and pre-swing was shown to be 19.5 %, 18.3 %, 41.5 %, and 20.7 %, respectively. Meanwhile, when the sub-threshold stimulus was applied, it was shown to be 21.2 %, 18.6 %, 38.4 %, and 21.8 %, respectively. Compared to gait without vibration stimulus, the proportion of the loading response and pre-swing decreased. In particular, when the sub-threshold stimulus was applied, the loading response and pre-swing decreased by 1.8 % and 1.7 %, respectively, which showed a high decline compared to the threshold stimulus.
When the threshold stimulus was applied to the Achilles tendon, the proportion of the loading response, mid stance, terminal stance, and pre-swing was shown to be 20.9 %, 17.4 %, 43.9 %, and 17.8 %, respectively. Meanwhile, when the sub-threshold stimulus was applied, it was shown to be 19.2 %, 15.9 %, 41.9 %, and 23.0 %, respectively.
Since the change in the gait cycle after threshold stimulus and sub-threshold stimulus application was similar, no significant difference in the changes was found according to the intensity of vibration stimulus.
Fig. 2The percentage of the gait event in the stance phase during the gait with each condition (AT_(S)TS: the (S)TS was applied to the Achilles tendon, TAT_(S)TS: the (S)TS was applied to the tibialis anterior tendon)
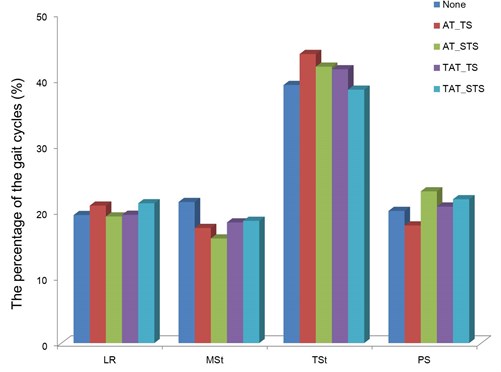
3.2. Comparison of muscle activity
The activity of the tibialis anterior and gastrocnemius according to characteristics of the vibration stimulus is presented in Fig. 3. The muscle activity was obtained by constructing a body model based on the marker data acquired by the three-dimensional motion analyzer, and then comparatively analyzed after setting the gait event.
When the threshold stimulus was applied to the tibialis anterior, the muscle activity was shown to be 0.39 mV in the tibialis anterior and 0.28 mV in the extensor digitorum longus, which showed a 14.1 % and 22.0 % increase, respectively, compared to gait without vibration stimulus. Meanwhile, when the sub-threshold stimulus was applied to the tibialis anterior, the muscle activity was shown to be 0.39 mV in the tibialis anterior and 0.29 mV in the extensor digitorum longus, which showed a 15.3 % and 29.4 % increase, respectively, compared to gait without vibration stimulus.
When the threshold stimulus was applied to the Achilles tendon, the muscle activity was shown to be 0.008 mV in the soleus and 0.07 mV in the gastrocnemius. Meanwhile, when the sub-threshold stimulus was applied to the Achilles tendon, the muscle activity was shown to be 0.02 mV in the soleus and 0.11 mV in the gastrocnemius, which were similar to the muscle activity of gait without vibration stimulus.
When the vibration stimulus was applied to the tibialis anterior, the activity of the tibialis anterior and extensor digitorum longus, the dorsiflexors, increased. Meanwhile, when the vibration stimulus was applied to the Achilles tendon, no significant difference in the activity of the soleus and gastrocnemius, and plantarflexors was found compared to gait without vibration stimulus. The increased activity of the tibialis anterior was similar between the threshold stimulus and sub-threshold stimulus, which showed no significant difference according to the intensity of vibration stimulus.
Fig. 3The change of muscle activity which was calculated by the root mean square
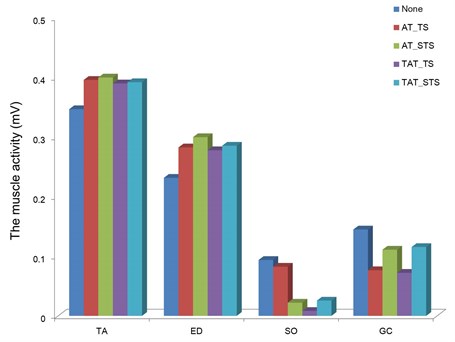
3.3. Comparison of the range of motion of the ankle
The changes in the range of motion of the ankle according to the characteristics of vibration stimulus are presented in Fig. 4. The range of motion of the ankle was also obtained by constructing the body model as shown in the comparison of muscle activity. The dorsiflexion and plantarflexion angles of the ankle during the stance phase were obtained based on force platform data, and then analyzed.
Fig. 4The changes in the dorsiflexion and plantarflexion angles of the ankle during the stance phase
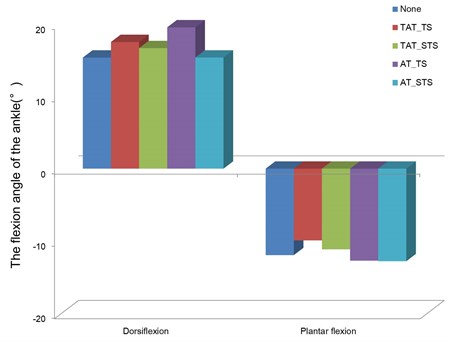
When the threshold stimulus was applied to the tibialis anterior, the dorsiflexion angle and the plantarflexion angle was shown to be 17.5° and 9.9°, respectively, which showed a 14 % increase in the dorsiflexion angle compared to gait without vibration stimulus. When the sub-threshold stimulus was applied to the tibialis anterior, the dorsiflexion angle and the plantar flexion angle was shown to be 16.6° and 11.1°, respectively, which demonstrated an 8.4 % increase in the dorsiflexion angle compared to gait without vibration stimulus.
Meanwhile, the dorsiflexion angle and the plantarflexion angle was shown to be 19.5° and 12.7°, respectively, when the threshold stimulus was applied to the Achilles tendon, and 15.3° and 12.8°, respectively, when the sub-threshold stimulus was applied to the Achilles tendon, which showed a 7 % increase in the plantarflexion angle compared to gait without vibration stimulus.
The dorsiflexion angle tended to increase when the vibration stimulus was applied to the tibialis anterior, whereas the plantarflexion angle tended to increase when the vibration stimulus was applied to the Achilles tendon. This pattern was associated with the pattern of muscle activity.
4. Discussion
This study was conducted to investigate if vibration stimulus whose characteristics were changed induces changes in gait pattern, and to comparatively analyze the changes in gait cycle and muscle activity.
When gait events such as heel contact (HC), heel off (HO), toe contact (TC), and toe off (TO) were detected using a footswitch mounted to the soles of the feet, and then the stimulus site was changed according to the gait event, the major changes were observed according to the application site of vibration stimulus. When the vibration stimulus was applied to the tibialis anterior, the proportion of the loading response and pre-swing in the stance phase decreased. When the vibration stimulus was applied to the Achilles tendon, the proportion of the threshold stimulus in the stance phase decreased. The body response variation depending on the application site of vibration stimulus was consistent with the result of a study conducted by Cholewiak et al. [12], and also consistent with the result of a study, conducted by Talis et al. [13], reporting that the stimulus directly applied to the tendon induced the body response over the wide range of the body. The changed gait cycle according to the application site of vibration stimulus is likely to be attributable to the direct involvement of vibration stimulus in the contraction of the muscle influencing gait cycle. Eklund et al. [14] reported that local vibration stimulus induced reflective muscle contraction. Ribot-Ciscar et al. [15] reported that short vibration stimulus caused the continuous occurrence of involuntary muscle contraction.
In gait event, the tibialis anterior is significantly activated to increase the effectiveness of the heel rocker which is performed for body center shift during the loading response phase, and the soleus and gastrocnemius are significantly activated during the pre-swing phase to produce torque that is required for shifting the pressure center by lifting the heel that supports the body center in order to enter into the pre-swing phase [16]. As vibration stimulus applied to the tibialis anterior during the loading response phase promotes tibialis anterior contraction, and vibration stimulus applied to the Achilles tendon during the pre-swing phase promotes gastrocnemius contraction, it seems that torque generation that is required for the gait event is prematurely completed, leading to the shortening of gait event time. However, in the case of gait event such as mid stance phase where the activity of the ankle is regulated by the simultaneous action of the antagonist muscle during gait, it is not clear if the same result is also obtained. Thus, it is necessary to identify the optimal characteristics of vibration stimulus, via which both muscles can be stimulated simultaneously. As the time of the gait event can be adjusted by changing the application site of vibration stimulus, changes in the gait pattern caused by the weakening of the muscles involved in gait could be returned to normal via the application of vibration stimulus. Thus, this method can be considered similar to methods such as a rehabilitation program [5], proprioceptive neuromuscular facilitation (PNF) [17], and functional electrical stimulation (FES) [4, 6] that aim towards muscle strengthening for efficient gait.
In this study, 4 types of vibration stimulus that vary in the application site and intensity of sensory stimulus were used to induce the change of the gait cycle via the application of vibration stimulus. The intensity of sensory stimulus was calculated based on the threshold acquired from the tibialis anterior and Achilles tendon considering individual variation. The changes in the gait cycle and muscle activity, and the changes in the flexion-extension angle of the ankle obtained in the study showed that vibration stimulus with intensity less than threshold induced a similar effect to that of vibration stimulus with intensity equal to threshold. This result is consistent with that of a study, conducted by Stephen et al. [18], where gait variation of the elderly was reduced by applying mechanical vibration with an intensity less than threshold to the soles, that of a study, conducted by Kouzaki et al. [19], where the continuous contraction of the plantar flexor was observed by applying electrical stimulation with an intensity less than threshold, and that of a study, conducted by a Priplata et al. [20], where posture stability was improved by applying subsensory mechanical noise with an intensity less than threshold to the soles. Exogenous stimulus that are used for the recovery of body functions in a clinical practice may cause discomfort, and even has a possibility of acting as negative factors that disturb the sensory system [21]. Thus, a further study is required to support the utilization of stimulus with intensity less than threshold.
5. Conclusion
This study was conducted to analyze the changes in the gait cycle and muscle activity after applying vibration stimulus, whose characteristics were changed via the realtime feedback of the gait event, to the body. The results are as follows:
(1) When the vibration stimulus was applied to the tibialis anterior, the proportion of the loading response and pre-swing in the stance phase decreased. Meanwhile, when the vibration stimulus was applied to the Achilles tendon, the proportion of the pre-swing in the stance phase decreased. This result showed that the gait cycle was changed according to the application site of vibration stimulus.
(2) When the vibration stimulus was applied to the tibialis anterior, the activity of the tibialis anterior and extensor digitorum longus increased, which showed that the activity of the muscles used for gait was changed according to the application site of vibration stimulus.
(3) When the vibration stimulus was applied to the tibialis anterior, the dorsiflexion angle of the ankle increased. Meanwhile, when the vibration stimulus was applied to the Achilles tendon, the plantarflexion angle of the ankle increased. This result showed that the pattern of ankle movement was changed according to the application site of vibration stimulus.
(4) The changes in the gait cycle and muscle activity, and the changes in the flexion-extension angle of the ankle obtained in the study showed that vibration stimulus with intensity less than the threshold induced a similar effect to that of vibration stimulus with an intensity of threshold, and that no significant difference in the changes was found according to the intensity of vibration stimulus.
(5) Mechanical vibration stimulus that causes somatosensory stimulus affected the gait pattern via the adjustment of the characteristics of vibration stimulus, and even locally applied stimulus affected the broad part of the body.
In conclusion, changes in gait pattern can be induced via the adjustment of the application site and time, and vibration stimulus can be effectively used for the correction and rehabilitation of individual specific gait. In addition, the results of this study can be used as basic data for inferring the correlation of the characteristic of vibration stimulus with gait variation in gait rehabilitation using vibration stimulus.
References
-
Rueterbories J., Spaich E. G., Larsen B., Andersen O. K. Methods for gait event detection and analysis in ambulatory systems. Medical Engineering & Physics, Vol. 32, Issue 6, 2010, p. 545-552.
-
Boulic R., Magnenat-Thalmann N., Thalmann D. A. Global human walking model with real-time kinematic personification. The visual computer, Vol. 6, Issue 6, 1990, p. 344-358.
-
Hodt-Billington C., Helbostad J. L., Vervaat W., Rognsvag T., Moe-Nilssen R. Changes in gait symmetry, gait velocity and self-reported function following total hip replacement. Journal of Rehabilitation Medicine, Vol. 43, Issue 9, 2011, p. 787-793.
-
Nightingale E. J., Raymond J., Middleton J. W., Crosbie J., Davis G. M. Benefits of FES gait in a spinal cord injured population. Spinal Cord, Vol. 45, Issue 10, 2007, p. 646-657.
-
Baer G. D., Ashburn A. M. Trunk movements in older subjects during sit-to-stand. Archives of Physical Medicine and Rehabilitation, Vol. 76, Issue 9, 1995, p. 844-849.
-
Seifart A., Unger M., Burger M. Functional electrical stimulation to lower limb muscles after botox in children with cerebral palsy. Pediatric Physical Therapy, Vol. 22, Issue 2, 2010, p. 199-206.
-
Humphries B., Warman G., Purton J., Doyle T. L. A., Dugan E. The influence of vibration on muscle activation and rate of force development during maximal isometric contractions. Journal of Sports Science and Medicine, Vol. 3, 2004, p. 16-22.
-
Galica A. M., Kang H. G., Priplata A. A., D’Andrea S. E., Starobinets O. V., Sorond F. A., Cupples L. A., Lipsitz L. A. Subsensory vibrations to the feet reduce gait variability in elderly fallers. Gait Posture, Vol. 30, Issue 3, 2009, p. 383-387.
-
Gescheider G. A., Bolanowski S. J., Pope J. V., Verrillo R. T. A four-channel analysis of the tactile sensitivity of the fingertip: frequency selectivity. Spatial summation, and temporal summation. Somatosensory and Motor Research, Vol. 19, Issue 2, 2002, p. 114-124.
-
So H. J., Oh H. Y., Kim S. H., Kim D. W. A study on estimated sub-threshold intensities of somatosensory stimuli based on somatosensory evoked potentials of EEG. Third International Conference on ISDEA, 2013, p. 190-194.
-
O’Connor C. M., Thorpe S. K., O’Malley M. J., Vaughan C. L. Automatic detection of gait events using kinematic data. Gait Posture, Vol. 25, Issue 3, 2007, p. 469-474.
-
Cholewiak R. W., Brill J. C., Schwab A. Vibrotactile localization on the abdomen: Effects of place and space. Perception & Psychophysics, Vol. 66, Issue 6, 2004, p. 970-987.
-
Talis V. L., Solopova I. A. Vibration-induced postural reaction continues after the contact with additional back support. Motor Control, Vol. 4, Issue 4, 2000, p. 407-419.
-
Eklund G., Hagbarth K. E. Normal variability of tonic vibration reflexes in man. Experimental Neurology, Vol. 16, Issue 1, 1966, p. 80-92.
-
Ribot-Ciscar E., Roll J. P., Gilhodes J. C. Human motor unit activity during post-vibratory and imitative voluntary muscle contractions. Brain Research, Vol. 716, Issue 1, 1996, p. 84-90.
-
Perry J. Gait analysis normal and pathological function. U.S.A., 1992.
-
Rees S. S., Murphy A. J., Watsford M. L., McLachlan K. A., Coutts A. J. Effects of proprioceptive neuromuscular facilitation stretching on stiffness and force-producing characteristics of the ankle in active women. Journal of Strength and Conditioning Research, Vol. 21, Issue 2, 2007, p. 572-577.
-
Stephen D. G., Wilcox B. J., Niemi J. B., Franz J. R., Kerrigan D., D'Andrea S. E. Baseline-dependent effect of noise-enhanced insoles on gait variability in healthy elderly walkers. Gait & Posture, Vol. 36, Issue 3, 2012, p. 537-540.
-
Kouzaki M., Kimura T., Yoshitake Y., Hayashi T., Moritani T. Subthreshold electrical stimulation reduces motor unit discharge variability and decreases the force fluctuations of plantar flexion. Neuroscience Letters, Vol. 513, Issue 2, 2012, p. 146-150.
-
Priplata A. A., Patritti B. L., Niemi J. B., Hughes R., Gravelle D. C., Lipsitz L. A., Veves A., Stein J., Bonato P., Collins J. J. Noise-enhanced balance control in patients with diabetes and patients with stroke. Annals of Neurology, Vol. 59, Issue 1, 2006, p. 4-12.
-
Simeonov P., Hsiao H., Powers J., Ammons D., Kau T., Amendola A. Postural stability effects of random vibration at the feet of construction workers in simulated elevation. Applied Ergonomics, Vol. 42, Issue 5, 2011, p. 672-681.
About this article
This research was supported by Basic Science Research Program through the National Research Foundation of Korea (NRF) funded by the Ministry of Education, Science and Technology (2012R1A2A2A01012599) and this paper was supported by research funds of Chonbuk National University in 2012.