Abstract
Dislocation avalanches and strain bursts in the boards of radio-engineering equipment were investigated. For that purpose a cascade of navigation devices boards was installed on the vibration stand and experiments were performed in the 0.5 – 10 Hz vibration range at 0 – +45 °C temperature. Amplitude method was applied to determine the coordinates of localized sources of acoustic emission and research of mechanical stress in local points of material was performed using axial compression tests on tensile machine. The results indicate the initial increase in tension and relative deformation and further their decrement. The acquired experimental data on acoustic emission reflect the formation of microcracks and the instability of mechanical tension, its avalanche and explosive tendency in the material when the micro volumes of material are torn.
1. Introduction
Constant mechanical stress at the mounting points of most of the radio-engineering electronic products increases due to exploitation and depends on the work environmental conditions and materials the board is made from, usually composite materials and plastics. During installation of the board and exploitation either in normal or extreme conditions the board is subjected to vibration, temperature and pressure changes resulting in the occurrence of plastic deformations due to movement of lattice dislocations.
In microcrystals that were plastically deformed inner dislocation avalanches influence the occurrence of mechanical stress spike - bursts. Dislocation avalanches is a unique feature that does not depend on the specific properties of material and it cannot be avoided by controlling the path of deformation or other crackling noise phenomena [1], such as Barkhausen noise, that is emitted along the hysteresis loop in ferromagnetics [2] or metalelectrics [3], acoustic emission during fracture [4].
The distribution of mechanical stress during dislocation avalanches and its dependence on microcrystal size can be investigated by combining the three-dimensional simulations of the dynamics of interacting dislocations with statistical analysis of corresponding behavior [5]. Several specific dislocations become geometrically unattached during the same case, which demonstrates the importance of long elastic interactions in the initiation of mechanical stress burst. The avalanche has a strongly anisotropic shape with more than 60 % of the deformation occurring on one of the four equivalent sets of slip planes. The statistical analysis of the avalanche distribution suggests that the fractal dimension of the avalanches is close to two, indicating an effective lamellar shape [6].
The existence of unstable bursts in the plastics due to mechanical stress was found [7, 8]. Dislocation avalanches in the polycrystals are limited by the grain boundaries and this can lead to a noticeable smoothing of deformation. The polymeric composite materials with epoxy matrix are used for navigation devices boards which work in various conditions of radiation and vibration. The monitoring the form of dislocations before the microcracks occur in the material of the board during exploitation has to be realized. For these purposes grids of micropiezosensors are useful and mechanical stress accumulation in the microlayers can be measured [9-11]. The goal of this work is the investigation of dislocation avalanches and strain bursts in the boards of radio-engineering equipment operated under heavy experimental condition.
2. Methods and specimens
The investigation of influence of vibrations on the material of the board of radio-engineering equipment can be done using laboratory facilities consisting of source of vibration, transducers, signal registering and data processing apparatus. A cascade of navigation devices boards was installed on the vibration stand as shown in Fig. 1. The tension and compression of the upper and lower layers of the printed circuit boards were taken using the camera.
Fig. 1Cascade of boards on the vibration stand
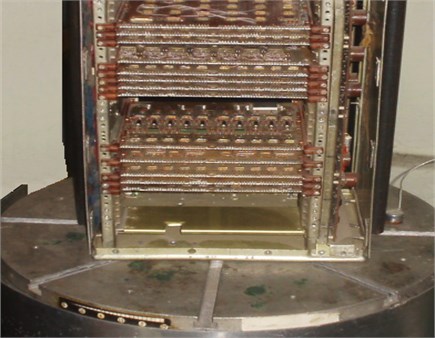
Fig. 2The experiments with modernised differential-phase scanning laser mіcroscope
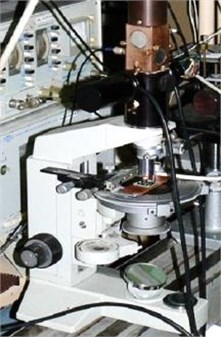
The possibility of controlling the form of dislocations before the microcracks occur in the material of the board during exploitation was tested. The microfractures due to Portevin-Le Chatelier PLC effect and microdefects were monitored using modersinsed differential-phase scanning laser microscope that is shown in Fig. 2. Then, based on the data from 8 localities on the board the initial length of microelement () and the length after deformation () were used in the evaluation . The data of increase of mechanical stresses was determined using the methodology of smoothing of mechanical stress accumulation in the microlayers and is shown in Fig. 3. The data point were widely scatered along the vertical axis , therefore the points were connected for data averaging. The signals and noises of different origin can be occurred during local plastic deformation as signals of the friction between the board and mounting points on the stand, the intrinsic noise of the test stand, etc. These factors make the acquisition of data more difficult. This is especially important for devices, which are sensitive to acoustic emission. Therefore for discrete signals various filtering methods are used like discrimination of sources according to location [4], spectral composition [5].
Experiments were performed in the 0.5 – 10 Hz vibrations range; 0 – 3 V voltage range; 0 – +45 °C temperature range. The phenomenon of acoustic emission occurs when the restructuring of deformed structures at different scale levels takes places. During mechanical stress relaxation elastic energy in the deformed regions of material is being emitted in the form of acoustic emission waves of different frequency and amplitude. This is can be detected by diagnostic equipment. In addition, the acoustic signal can provoke changes in material structure and influence the self-organization of defects. The amplitude method was used for determination of the coordinates of localized acoustic emission sources. This method is based on the dependence of the attenuation of acoustic wave on the distance between the source and the acoustic transducer. Acoustic emission signal was detected with experimental equipment shown in Fig. 4. Sensor for conversion of acoustic vibrations of the specimen into voltage change, filters for filtration of the acoustic wave signal from the noise and external activity of the environment, amplifiers of the acoustic wave signal to the 1 – 3 V level were implemented into experimental facilities. The shape of registered signal is shown in Fig. 5. The signal/noise radio is acceptable to carry out the determination of the place of the source of acoustic emission on the electronic board. The accuracy of about 3 – 5 % for determining the coordinates of sources can be achieved.
Fig. 3The diagram of increase of mechanical stresses in the microlayers of material
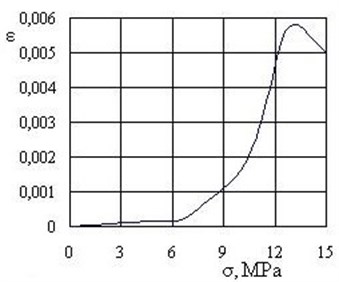
Fig. 4Acoustic emission signal detection and data processing facilities
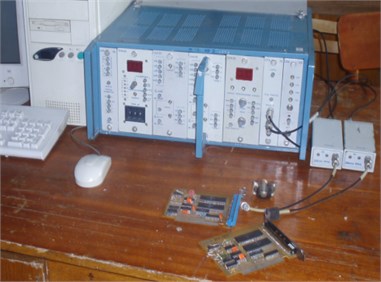
Fig. 5Oscillographic registration of acoustic emission signal and determination of signal parameters: where DA – the difference in the arrival time of signals and its subsequent self-smoothing due to piezoeffects, A max – amplitude of oscillation
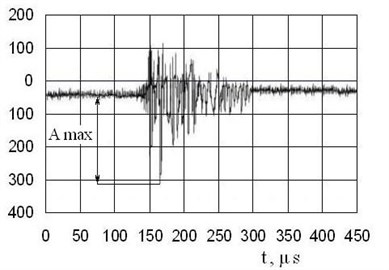
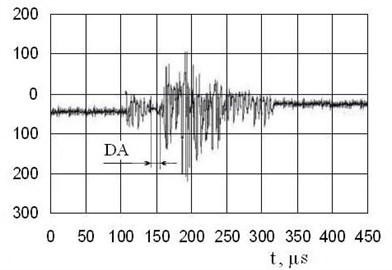
2.1. Experimental results
Depending on the offset of the local points the actual rupture of the microlocality happens (Chernov-Luders lines), which implies that the microfibers of the composite board are destroyed. The data was compared with the tensile testing machine.
The tensile testing machine IR 5057-50 and samples for axial compression were used for investigation of mechanical stresses in the local points of boards from polymeric composite materials with epoxy matrix. The machine was improved by implementation of spring dynamometer, which was calibrated for micron movement during the process of tension-compression. Results of “smoothed” acoustic spectra of local plastic deformation in different temperature are shown in Fig. 6. Mechanical stress and relative deformation of investigated samples are shown in Fig. 7.
Fig. 6Acoustic spectra of microsensors on local points on the board: a – “smoothing”; b – reduction in avalanche formation and stabilization of difference of acoustic emission (after 10 s); where 1 – +45 °C; 2 – +20 °C; 3 – +8 °C; 4 – 0 °C
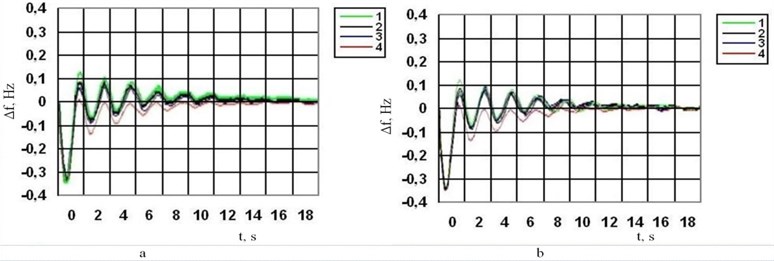
Fig. 7Mechanical stress and deformation curves: a – stress in the material of the board; b – the compression diagram. Curves correspond to the temperature values on Fig. 7
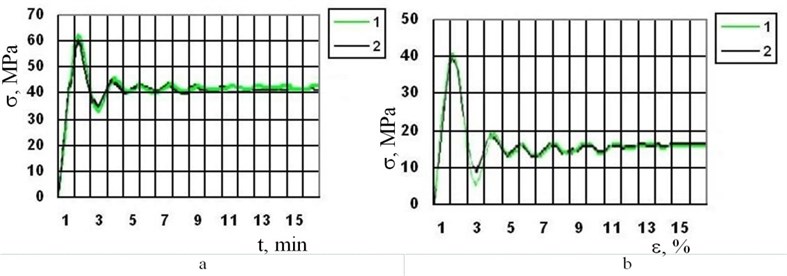
The results indicate the initial increase of mechanical stress and relative deformation and further their decrement. In the region [0; 57] MPa mechanical tension is practically linear and depends on the relative deformation. The limit of proportionality 20 MPa (correspond to the relative deformation value of 6·10-4) is achieved when time value 1.2 min. Reduction of growth rate of mechanical stress when is due to destruction of material structure. The receiving piezo converter and a waveguide which was in acoustic contact with the sample were used during experiments. The data was stored in the memory of personal computer and is shown in Fig. 8.
From Fig. 8 it can be seen, that when the plastic deformation at the yield plateau is realized by oncoming movement from piezoeffect of two Chernov-Luders bands, the localization of acoustic emission sources exhibits similar characteristics as was found for well examined samples of low-carbon steel. The data acquired in this work differ only by the order of mechanical stress, but doesn’t negate the established pattern of the acoustic emission signals occurring when local points are subjected to deformation. In this case, the motion of the first Chernov-Luders line corresponds to the spatial distribution of acoustic emission sources. The second Chernov-Luders line is moving towards the first and the velocity of lines movement is dropped to zero. This means complete lack of resistance due to complete destruction of the material in the local point, where it was a microcrack filled with air developed. This is confirmed by the fronts of acoustic emission pulses. Then destruction of the neighboring local points begins as can be observed in Fig. 7 after 120 seconds from the beginning of tension-compression of the sample.
Fig. 8AE activity when sample is being deformed (dashed lines mark characteristic stages: I – elastic, II – yield plateau, III – “silence zone”, IV – stage of parabolic hardening)
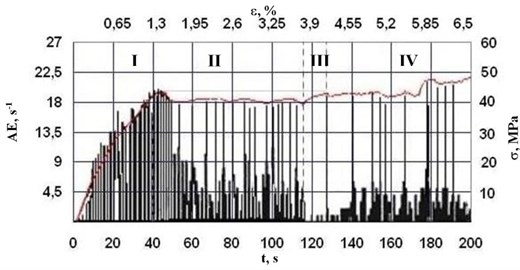
3. Conclusions
Dislocation avalanches and strain bursts in the boards of radio-engineering equipment operated under heavy experimental condition have been investigated experimentally using vibration stand, grid of piezotransducers, data processing and registration facilities. The acquired experimental data on acoustic emission reflects the formation of microcracks and the instability of mechanical tension, its avalanche and explosive tendency in the material when the microvolumes of material are torn. The phenomenology of these bursts of mechanical stress closely resembles the phenomenology of macroscopic instability of plastics. Time disruptions are associated with spatial localization because each burst of mechanical stress corresponds to the formation of a narrow slip line or slip band as it is discussed in work. Time-spatial localization of deformation is associated with instability of plastics and has negative affect on the formation characteristics. A classic example is the Portevin - Le Chatelier (PLC) effect [12] – the bursts of mechanical stress that result because of the interaction between dislocations and distribution solutions. The kinetics of the burst and avalanche-like increase of boards microdefects is similar to the disruption of the latent period of the phase formation in polycrystals under vibration or wave oscillations of different frequencies and piezoelectric effects in local points. Herewith in one microlayer a positive charge is formed and a negative charge in the neighboring layer, which are self-balancing and reduce the amplitude of the forming avalanche. The instability during the microcracks formation in the polymeric composite boards can be avoided if the right path of the process is selected. The temperatures and mechanical stress values when dislocations and the velocity of their spreading are of the same order of magnitude must be avoided in such case. Apart from that, larger mechanical stress fluctuations can disturb the controlling of the resulting shape during the plastics forming process.
References
-
Sethna J. P., Dahmen K. A., Myers C. R. Crackling noise. Nature, Vol. 410, 2001, p. 242-250.
-
Durin G., Zapperi S. The Barkhausen effect. The Science of Hysteresis, Vol. 2, 2006, p. 181-267.
-
Colla E. V., Chao L. K., Weissman M. B. The Barkhausen noise in the relaxor ferroelectric. Physical Review Letters, Vol. 88, No. 1, 017601, 2002, p. 1-4.
-
Salminen L. I., Tolvanen A. I., Alava M. J. Acoustic emission from paper fracture. Physical Review Letters, Vol. 89, No. 19, 185503, 2002, p. 1-4.
-
Uchic M. D., Dimiduk D. M., Florando J. N., Nix W. D. Sample dimensions influence strength and crystal plasticity. Science, Vol. 305, No. 5686, 2004, p. 986-989.
-
Csikor F. F., Motz C., Weygand D., Zaiser M., Zapperi S. Dislocation avalanches, strain bursts and the problem of plastic forming at the micrometer scale. Science, Vol. 318, No. 5848, 2007, p. 251-254.
-
Tinder R. F., Trzil J. P. Millimicroplastic burst phenomena in zinc monocrystals. Acta Metallurgica, Vol. 21, Issue 7, 1973, p. 975-989.
-
Potthoff H. H. Velocities of dislocation groups in very thin neutron-irradiated copper single crystals measured by slip line cinematography. Physica Status Solidi (A), Vol. 77, Issue 1, 1983, p. 215-224.
-
Dimiduk D. M., Woodward C., Le Sar R., Uchic M. D. Scale-free intermittent flow in crystal plasticity. Science, Vol. 312, No. 5777, 2006, p. 1188-1190.
-
Miguel M. C., Vespignani A., Zapperi S., Weiss J., Grasso J. R. Interrmitted dislocation flow in viscoplastic deformation. Nature, Vol. 410, 2001, p. 667-671.
-
Voronov S., Poplavko Y., Bogorosh A., Bubulis A. Piezo- and pyroelectric GaAs sensors integrated in one crystal with GaAs FET. Journal of Vibroenergineering, Vol. 12, Issue 1, 2010, p. 119-123.
-
Kubin L. P., Fressengeas C., Ananthakrishna G. Collective behaviour of dislocations in plasticity. Dislocations in Solids, Vol. 11, 2002, p. 101-192.
About this article
This research was supported by the Lithuanian State Science and Studies Foundation Contract No. V-09060.