Abstract
This paper mainly studies the mechanism and efficiency of ammonia-nitrogen removal from circulating sewage in closed fish tank system. According to some factors affecting the removal efficiency (ultrasonic power, ultrasonic time and water oxygen concentration). Simulated circulating sewage mixed with oxygen through a venturi tube and then entered a closed experiment box for ultrasonic experiments. Finally, the removal efficiency was determined by Knott's reagent photometry. In the experiment, the PH value of the simulated sewage was 7.5, the indoor water temperature was 20 °C, and the free ammonia was selected as 0.02 mg/L (total ammonia-nitrogen was 1.6 mg/L) according to the standard. The experiment found that when the ultrasonic power is 400 W, the action time is 2 h, and the oxygen concentration of water is 23.8 mg/L, the efficiency of removing ammonia-nitrogen in water is about 65 %. When applied on fishing vessels, increasing the power can increase the removal efficiency.
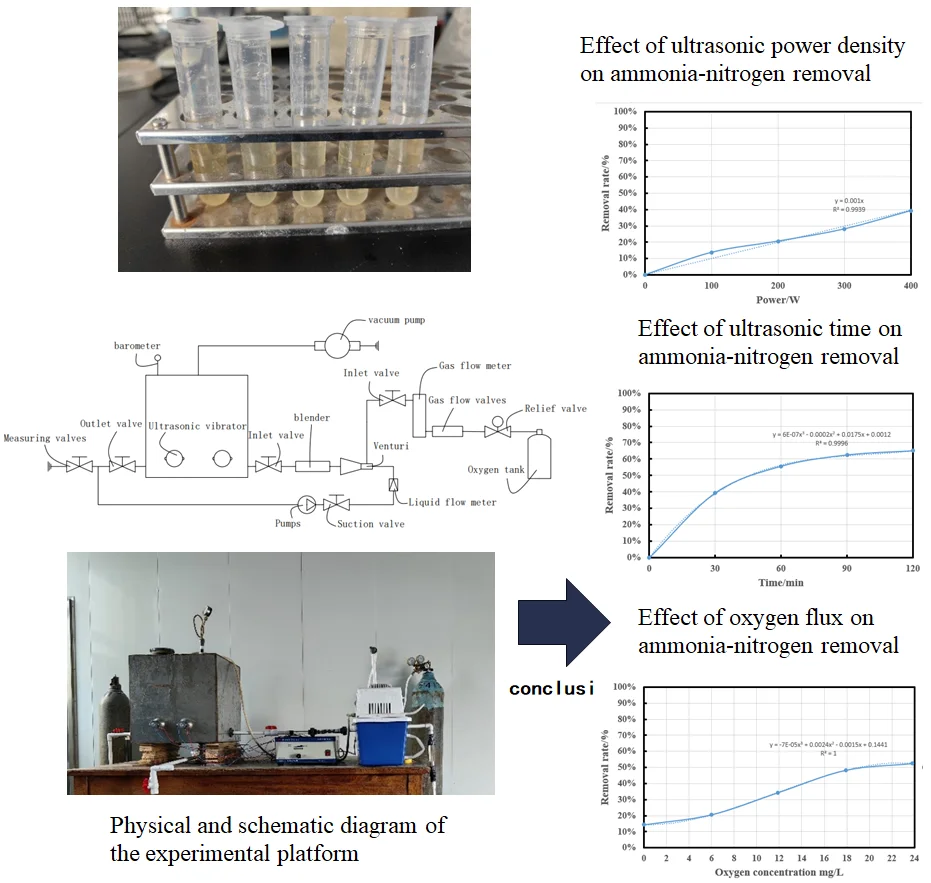
Highlights
- Treatment of low-concentration aquaculture effluent by ultrasonic vibration.
- Effect of effluent oxygen content on removal efficiency in ultrasonic vibration.
- The small experimental platform simulates the aquaculture sewage treatment process.
1. Introduction
In the transport and culture of fish, ammonia-nitrogen is mainly derived from nitrogen waste produced during the metabolism of fish. In water, urea will gradually decompose into NH3 and CO2, which NH3 is one of the main pollutants [1]. The presence of too much ammonia-nitrogen in the water will have a certain toxic effect on the fish. When the concentration of ammonia-nitrogen in the water exceeds a certain range, ammonia-nitrogen stimulates the secretion of ammonia oxidase on the gills of fish, converting ammonia into nitrite and nitrate and other nitrogen-based substances [6]. These substances have certain toxicity, can destroy fish body cell tissue, affect fish respiration and other functions, resulting in the death of fish. Experiments have shown [7] that excessive ammonia-nitrogen in water will inhibit the enhancement of fish immunity, reduce the resistance of fish to pathogens. Therefore, excessive ammonia-nitrogen content is a common predisposition factor to fish diseases [4].
At present, the most commonly used treatment technologies for ammonia-nitrogen removal in water include biological treatment [5], chemical precipitation [6], air stripping [7], microwave radiation, breakpoint chlorination, membrane filtration and adsorption [8].
Ultrasonic technology is to generate high-frequency mechanical waves in water, so that the attraction between liquid phase molecules is broken to form cavitation nuclei, which can promote the removal and decomposition of ammonia nitrogen. [9]. These compression and sparse waves are generated and disappear in the water in an instant, accompanied by tiny vacuum air bubbles. The lifetime of cavitation nuclei is about 0.1 nanoseconds, and it can produce a local high temperature and pressure environment at the moment of explosion. Produces a fast and intense impact microjet called ultrasonic cavitation [10-12]. At this time, part of the ammonia molecules are adsorbed to the vacuum bubble and catalyzed oxidation at the bubble wall to generate nitrogen [13].
2. Materials and methods
In this study, 0.02 mg/L free ammonia was selected as the standard to prepare ammonia-nitrogen solution by selecting the fishery standard as the reference basis, and the water temperature was set to 20 °C. Ammonia-nitrogen removal efficiency is achieved by applying a (0-400 W) ultrasonic generator combined with a vibrator to create a small simple model platform. The ultrasonic cycle was selected (0-2 hours), different oxygen concentrations (0-1 L/min) were selected, and the removal efficiency under different conditions was recorded. The main objective of the study was to evaluate the efficiency of ultrasonic technology in removing ammonia-nitrogen from sewage.
2.1. Materials and chemicals
The NH4Cl was dissolved in tap water (tap water PH was determined to be 7.54, total ammonia concentration content was about 0.02 mg/L), and the nonionic ammonia concentration was controlled at 0.02 mg/L. In addition, by adding an appropriate H₂SO₄ solution, the pH value of the solution becomes 7.5.
It can be seen from Table 1 that when the PH value is 7.5 and the temperature is 20 °C, when the nonionic ammonia in the water is 0.02 mg/L, the total concentration of ammonia is about 1.6 mg/L.
Table 1Total ammonia (NH4++NH3) concentration, of which nonionic ammonia concentration is 0.020 mg/L
Temperature / ℃ | PH | Percentage of nonionic ammonia / % |
20 | 7.0 | 5.1 |
20 | 7.5 | 1.6 |
20 | 8.0 | 0.52 |
20 | 8.5 | 0.18 |
Fig. 1Experimental preparation materials
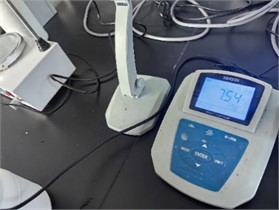
a) Tap water pH
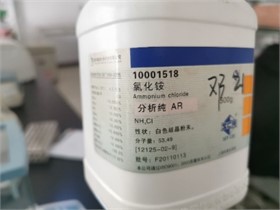
b) Ammonium chloride
2.2. Experimental setup
The experimental platform is a small simulation platform that simply simulates the ultrasonic treatment process of circulating sewage. The ultrasonic device in the experimental setup is a laboratory-sized sonic device (Haineretc, HNG-FP-II, 900 W, 28 kHz), which is connected to four 100 W vibrators by an ultrasonic generator, and the vibrators are glued with screws and special glue on the outer wall of the experiment box (the thickness of the chamber is about 5 mm). The power input can be adjusted from 0 to 900 W. The upper outlet of the experimental chamber is connected to the vacuum pump, which is conducive to the removal reaction of the separated gas in the water body in the experiment box. Before the circulating water enters the experiment box, the oxygen concentration in the water is increased through the venturi tube, and the temperature in the experiment box is maintained through the continuous circulation of water. The ultrasonic test is performed at a constant temperature of 20±2 °C. The detailed experimental setup is shown in Fig. 2.
Fig. 2Physical and schematic diagram of the experimental platform: 1 – ultrasonic vibrator; 2 – barometer; 3 – vacuum pump; 4 – inlet valve; 5 – blender; 6 – venturi; 7 – inlet valve; 8 – gas flow meter; 9 – gas flow valves; 10 – relief valve; 11 – oxygen tank; 12 – liquid flow meter; 13 – suction valve; 14 – pumps; 15 – outlet valve; 16 – measuring valves
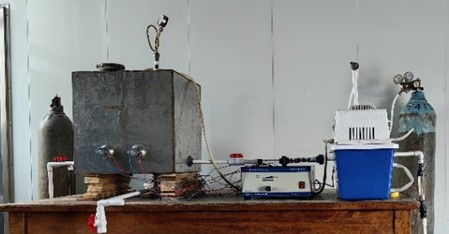
a)
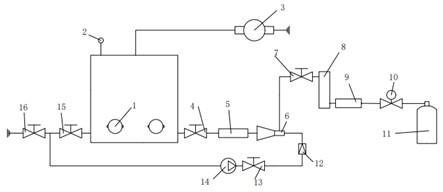
b)
2.3. Experimental procedures
Turn on the water pump by closing the left outlet valve, and allow the solution to complete circulation throughout the experimental platform. The removal efficiency of ammonia nitrogen at different times, different powers and different oxygen concentrations was tested by the control variable method, and finally the removal efficiency was determined by Knott's reagent photometry.
3. Experimental results and discussion
Ultrasonic power density, sonication time, and oxygen concentration will all affect the efficiency of ammonia-nitrogen removal to a certain extent. Therefore, the ammonia-nitrogen removal experiment was tested according to the control variable method for various influencing factors.
3.1. Effect of ultrasonic power on ammonia-nitrogen removal efficiency
Ultrasound has two removal mechanisms, including methods of free pyrolytic degradation and oxidation. Ultrasonic power density is the main factor in ammonia-nitrogen in ultrasonic treatment solutions. The effect of ultrasonic power on ammonia-nitrogen removal is obvious. The ultrasonic influence test of this experiment was carried out at pH 7.5 for 2 h, the oxygen concentration was 16.7 mg/L (flow rate was 0.7 L/min), and the water circulation speed was 100 L/h. The ultrasonic powers for ammonia-nitrogen removal were set to 100, 200, 300, and 400 W, respectively, and the removal efficiencies obtained by Knott's photometry were 13.75 %, 20.63 %, 28.13 %, and 39.38 %, respectively (Fig. 3). These results show that the higher the ultrasonic power density, the more effective it is to blow ammonia-nitrogen from water. Because of the pyrolysis reaction, the ultrasonic power density increases the number and density of cavitation bubbles in the solution. Therefore, the increase in ultrasonic power can be applied to more complex and concentrated contaminated waters. The ultrasonic power selected for this experiment is small. However, this study shows that higher ammonia-nitrogen removal efficiency can be achieved at pH 7.5 and higher ultrasonic power. At the same time, the specific ultrasonic treatment cost will also be reduced due to the shorter ultrasonic treatment time, and in this way, ultrasonic treatment can be a technical and economical option in wastewater treatment. The ammonia-nitrogen removal rate can be simplified as a function of ultrasonic power, and the two increase almost linearly.
3.2. Effect of sonication time on ammonia-nitrogen denitrification efficiency
Sonication time is also an important factor affecting the removal efficiency, ultrasonic testing is performed at constant temperature (20 °C), 400 W ultrasonic power and pH 7.5 and oxygen concentration 16.7 mg/L (flow rate of 0.7 L/min), water circulation speed of 100 L/H is a fixed value, time as a control variable. The ammonia-nitrogen removal efficiency of the solution was compared by controlling different ultrasonic times. The following compares the solubilities obtained by ultrasound of the solution within 30, 60, 90 and 120 minutes, respectively. It was found that the ammonia-nitrogen removal efficiency was 39.38 %, 55.63 %, 62.5 % and 65 %, respectively. But after the time reaches a certain value, the uptrend decreases significantly. The ammonia-nitrogen removal rate is simplified as a function of ultrasonic time (Fig. 4). The number of cavitation bubbles and the acoustic energy value of the solution increase with the increase of sonication time, it should be noted that the water temperature will increase when the ultrasonic test is performed during a long sonication time, and the increase of water temperature will bring about changes in various factors (PH, gas solubility, number of bacterial colonies in the solution, etc.) It is necessary to control the experimental impact caused by the rise of water temperature. Therefore, the sonication time achieved here has significant ammonia-nitrogen removal performance.
Fig. 3Effect of ultrasonic power density on ammonia-nitrogen removal
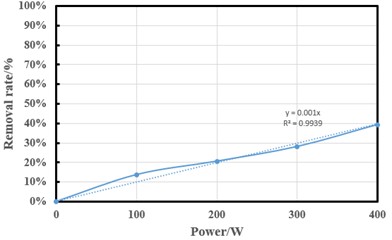
Fig. 4Effect of ultrasonic time on ammonia-nitrogen removal
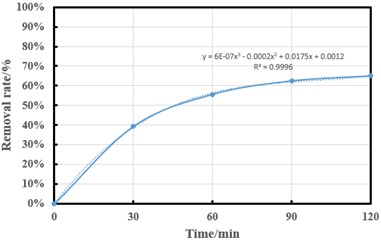
3.3. Effect of oxygen concentration in water on ammonia-nitrogen denitrification efficiency
Oxygen introduction is a relatively new method compared to various previous ultrasonic experiments to remove ammonia-nitrogen. Ultrasonic test is performed at constant temperature (20 °C), 400 W ultrasonic power, pH 7.5 and ultrasonic time is 2 h, the cycle speed is 100 L/H is a fixed value, and oxygen flux is the control variable to change the amount of oxygen dissolved in the water. By controlling the rate at which different oxygen enters the venturi tube, the ammonia-nitrogen removal efficiency is compared. Under the control of oxygen valve flow, the solution was sonicated at 0, 0.25, 0.5, 0.75 and 1 L/min oxygen flux (oxygen concentration of 0, 6, 11.9, 17.9 and 23.8 mg/L in water), respectively. Ammonia-nitrogen removal rate is calculated as a function ultrasonic cycle (Fig. 5). The use of Venturi gas-water mixer to mix oxygen with circulating water, so that the concentration of dissolved oxygen in the solution into the experimental chamber is much higher than the concentration of dissolved ammonia-nitrogen. At the same time the use of ultrasonic cavitation and Dalton’s law and Henry’s law to release ammonia-nitrogen dissolved in water. Oxygen is conducive to help the decomposition of free radicals in the ultrasonic reaction for strong redox action. It can accelerate the oxidation of ammonia-nitrogen and help reduce the concentration of ammonia-nitrogen in water. When the amount of oxygen dissolved is significantly greater than the amount of ammonia nitrogen dissolved in water, the dissolved ammonia nitrogen in the water is forced to be discharged. A vacuum valve is installed above the experiment chamber. The vacuum environment is conducive to the separation of gas and solution, so that ammonia nitrogen dissolved in the circulating water is released from the water. The experiments showed that the ammonia nitrogen removal efficiency was 14 %, 20.63 %, 34.38 %, 48.3 % and 52.5 %, respectively. The results showed that the trend began to slow down after the oxygen flux reached a certain value, and the upward trend and efficiency increased significantly when the oxygen concentration was 8-18 mg/L.
Fig. 5Effect of oxygen flux on ammonia-nitrogen removal
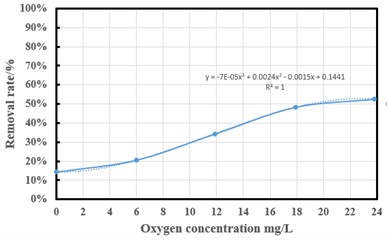
Fig. 6Ammonia nitrogen removal effect
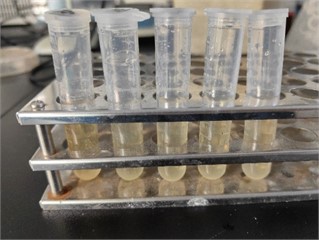
4. Conclusions
First, there are errors and uncertainties in the experiment. Failure to install real-time temperature monitoring may cause excessive temperature variations in the chamber. Second, the efficiency of the ultrasound may include other factors that may affect the experimental results.
The innovation of this paper: Compared with the ordinary ultrasonic removal of ammonia nitrogen in water, a venturi jet is added to this experiment to improve the removal efficiency of ammonia nitrogen by increasing the amount of oxygen dissolved in the water.
In this paper, the small platform simulation is mainly realized to remove ammonia-nitrogen from the sewage of fishing vessels at sea. There are many conditions affecting the removal efficiency, such as power density, ultrasonic time, oxygen concentration, and the efficiency of ammonia-nitrogen removal is mainly studied from these aspects. The main findings of the study are summarized below:
Ultrasonic cavitation is carried out at the same time, and oxygen is fused with water through a venturi jet, increasing the amount of oxygen dissolved in the water. Using Dalton and Henry's law and vacuum pump to separate the dissolved ammonia gas in the water, the purpose of removing the dissolved ammonia nitrogen in the water is achieved.
Experiments show that with the increase of ultrasonic power input, the removal efficiency is significantly improved. The ultrasound time increased and the efficiency was also obvious, but the rise rate decreased significantly after 60 min. The increase of oxygen flux increases the concentration of dissolved oxygen in water, which is more conducive to the removal of ammonia-nitrogen dissolved in water, but after reaching a certain value, the improvement efficiency is significantly reduced and begins to flatten.
In general, when the ultrasonic power is 400 W, the action time is 2 h, and the oxygen concentration of water is 23.8 mg/L, the efficiency of removing ammonia nitrogen in water is about 65 %. When applied on fishing vessels, increased power allows rapid removal of ammonia nitrogen in a short period of time. Compared to aeration, it saves more space in the hull.
References
-
B. Li et al., “Phosphorous recovery through struvite crystallization: Challenges for future design,” Science of The Total Environment, Vol. 648, pp. 1244–1256, Jan. 2019, https://doi.org/10.1016/j.scitotenv.2018.07.166
-
H. Shi, C. Li, L. Wang, W. Wang, J. Bian, and X. Meng, “In-situ synthesis of ZnSe nanoparticles onto monoclinic BiVO4 for boosted photocatalytic decomposition of ammonia nitrogen in water,” Journal of Environmental Chemical Engineering, Vol. 10, No. 6, p. 108881, Dec. 2022, https://doi.org/10.1016/j.jece.2022.108881
-
J. S. Salla, N. Padoin, S. M. Amorim, G. Li Puma, and R. F. P. M. Moreira, “Humic acids adsorption and decomposition on Mn2O3 and α-Al2O3 nanoparticles in aqueous suspensions in the presence of ozone,” Journal of Environmental Chemical Engineering, Vol. 8, No. 2, p. 102780, Apr. 2020, https://doi.org/10.1016/j.jece.2018.11.025
-
Y. Xu, Z. Lin, Y. Zheng, J.-P. Dacquin, S. Royer, and H. Zhang, “Mechanism and kinetics of catalytic ozonation for elimination of organic compounds with spinel-type CuAl2O4 and its precursor,” Science of The Total Environment, Vol. 651, pp. 2585–2596, Feb. 2019, https://doi.org/10.1016/j.scitotenv.2018.10.005
-
J. Wang, X. Quan, S. Chen, H. Yu, and G. Liu, “Enhanced catalytic ozonation by highly dispersed CeO2 on carbon nanotubes for mineralization of organic pollutants,” Journal of Hazardous Materials, Vol. 368, pp. 621–629, Apr. 2019, https://doi.org/10.1016/j.jhazmat.2019.01.095
-
C. Ma et al., “Typical layered structure bismuth-based photocatalysts for photocatalytic nitrogen oxides oxidation,” Science of The Total Environment, Vol. 855, p. 158644, Jan. 2023, https://doi.org/10.1016/j.scitotenv.2022.158644
-
H. Luo, Y. Zeng, D. He, and X. Pan, “Application of iron-based materials in heterogeneous advanced oxidation processes for wastewater treatment: A review,” Chemical Engineering Journal, Vol. 407, p. 127191, Mar. 2021, https://doi.org/10.1016/j.cej.2020.127191
-
N. Zhang, Y. Zhang, T. Bohu, S. Wu, Z. Bai, and X. Zhuang, “Nitrogen removal characteristics and constraints of an Alphaproteobacteria with potential for high nitrogen content heterotrophic nitrification-aerobic denitrification,” Microorganisms, Vol. 10, No. 2, p. 235, Jan. 2022, https://doi.org/10.3390/microorganisms10020235
-
J. Fang et al., “Characteristics of a novel heterotrophic nitrification-aerobic denitrification yeast, Barnettozyma californica K1,” Bioresource Technology, Vol. 339, p. 125665, Nov. 2021, https://doi.org/10.1016/j.biortech.2021.125665
-
K. Azis, S. Ntougias, and P. Melidis, “NH4+-N versus pH and ORP versus NO3--N sensors during online monitoring of an intermittently aerated and fed membrane bioreactor,” Environmental Science and Pollution Research, Vol. 28, No. 26, pp. 33837–33843, Jul. 2021, https://doi.org/10.1007/s11356-020-10534-4
-
J. S. Chang, M. N. Chong, P. E. Poh, J. D. Ocon, M. Z. H. Md Zoqratt, and S. M. Lee, “Impacts of morphological-controlled ZnO nanoarchitectures on aerobic microbial communities during real wastewater treatment in an aerobic-photocatalytic system,” Environmental Pollution, Vol. 259, p. 113867, Apr. 2020, https://doi.org/10.1016/j.envpol.2019.113867
-
Y. Sun et al., “Different acetonitrile degraders and degrading genes between anaerobic ammonium oxidation and sequencing batch reactor as revealed by stable isotope probing and magnetic-nanoparticle mediated isolation,” Science of The Total Environment, Vol. 758, p. 143588, Mar. 2021, https://doi.org/10.1016/j.scitotenv.2020.143588
-
W. Xing, Y. Wang, T. Hao, Z. He, F. Jia, and H. Yao, “pH control and microbial community analysis with HCl or CO2 addition in H2-based autotrophic denitrification,” Water Research, Vol. 168, p. 115200, Jan. 2020, https://doi.org/10.1016/j.watres.2019.115200
About this article
The authors have not disclosed any funding.
The datasets generated during and/or analyzed during the current study are available from the corresponding author on reasonable request.
The authors declare that they have no conflict of interest.