Abstract
The use of fossil fuels of all kinds, such as natural gas, coal and petroleum in energy production, is the main cause of environmental pollution from air, water and soil, which is the direct cause of acid rain, the destruction of forests, the acidity of lakes, and the extinction of many living creatures that could not resist what happened Burning this fuel is due to a change in the surrounding environment. From this, it becomes clear to us the need for new sources to produce clean energy that does not pollute the environment, and work must be developed on these new sources for use in the production of clean energy in the coming years. The purpose of this work is to determine the efficiency of vertical axis turbines to be among the components of a hybrid system (solar/wind) to generate electric power in the Kingdom of Saudi Arabia. The work seeks to answer the research question, what is the efficiency of vertical axis turbines in generating electricity within a hybrid system (solar/wind) to generate electric power in the Kingdom of Saudi Arabia. The aim of the work is to analyze the vertical axis turbines and determine efficiency within the hybrid system in the regions of Saudi Arabia. There are many renewable energy sources (wind, solar thermal energy, solar photovoltaic, biomass, small and large hydropower and geothermal energy). But their efficiency is not stable from time to time, so the idea of hybrid energy came to make up for the shortage, by integrating the energy source Renewables with one or more other sources of energy (In 1999, McGowan and Manwell (1999) presented a summary of WND-PV-DSL HPS progress in the United States.) whether it is a non-renewable or renewable source [1].
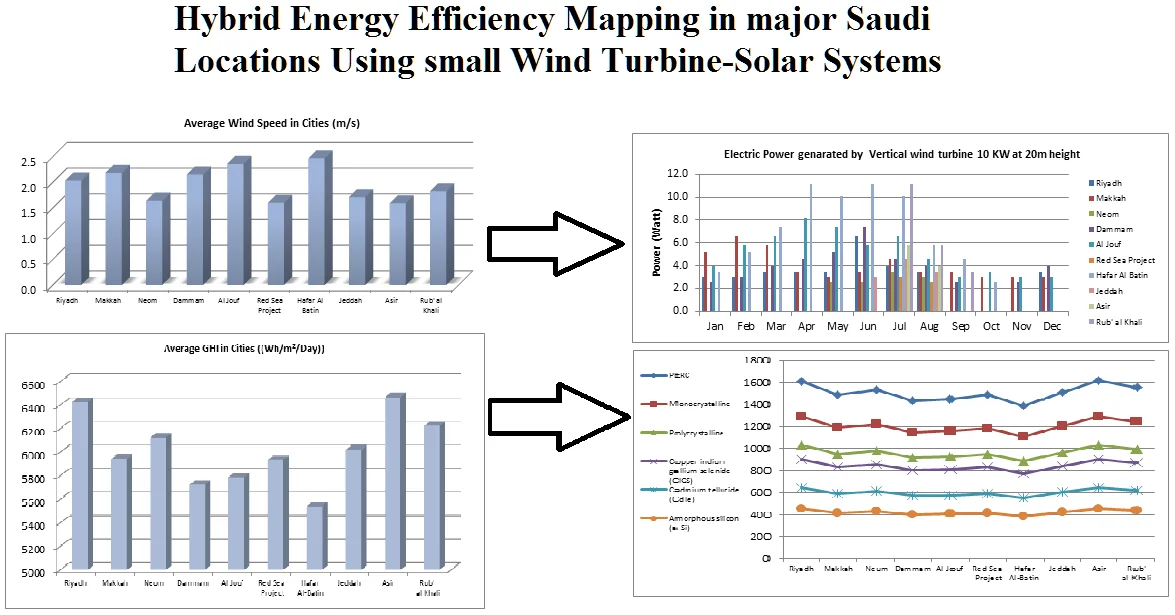
Highlights
- Small Wind Turbine-Solar Systems
- Hybrid Energy Efficiency Mapping in major Saudi Locations
- Renewable Energy in Saudi Arabia and Vision 2030
1. Introduction
1.1. Wind energy
Wind has kinetic energy that can be converted into electrical energy by turbines connected to fans of many types, and this type is abundant, renewable, widely distributed, clean, does not produce greenhouse gases emissions during operation, consumes no water, uses little land, and is less expensive than the physical aspect [2]. Wind energy can be classified into onshore and offshore according to the location of the facility. Offshore wind energy is characterized by stability, strength, and less visual impact, but the construction and maintenance costs are high [3]. Wind energy can be benefited from by connecting generators to the network, as is the case in large, medium, megawatt and even small systems that are usually separated from the network and used in isolated areas.
1.2. Solar energy
Solar energy is the cleanest source of energy, and it is transmitted in the form of electromagnetic radiation (or photons) of different wavelengths, of which it reaches the Earth for a period of 0.3-2.4 nanometer [4]. Solar energy can be converted into electrical energy by the following methods.
1.2.1. Solar cells (photovoltaic cells) (PV)
Each photon carries an amount of energy that it transfers completely to one of the electrons of the material falling on it, and if the frequency of the incident light is greater than the threshold frequency of the metal, then the electron is emitted from the surface of the metal, forming what is called the light current that is proportional to the intensity of the light (the number of photons) incident, this process is known as the photoelectric phenomenon [5].
1.2.2. Thermal conversion
Solar thermal energy is technologies that use heat energy from the sun directly to heat a carrier or heat carrier, which is most often water. The resulting hot water can be used for domestic and industrial purposes, and has virtually no carbon emissions because no fuel is burned to heat the water [6]. There are also advanced solar heat energy systems capable of trapping and collecting the sun’s energy to produce steam that is used to drive turbines to produce electricity.
1.2.3. A review of the concept of hybrid energy and examples of it
A hybrid energy system is a system that combines two or more energy sources. The need to think of such systems is to reduce cost and reduce dependence on fossil fuels, and to find environmentally friendly systems with high efficiency, examples of which are:
– Geothermal+solarPV.
– Biomass+solarCSP.
– SolarPV+fuelcells.
– Wind+solarPV.
– Biodiesel+wind.
– Gas+solarCSP.
– Coal+solar CSP [7].
Our study in this paper focuses on small hybrid power installations that can provide a capacity ranging from 5 watts to 30 watts, which consists of a turbine to generate energy by wind in addition to solar panels, and work has been done on ten different regions in the Kingdom of Saudi Arabia and a study The efficiency of five different wind turbines.
2. Wind energy in Saudi Arabia
2.1. Wind speed in Saudi Arabia
The wind speed in the Kingdom ranges from (1.2-22.1) m/s and at a rate of 2.1 m/s at the standard height, and Table 1 shows the values of wind speed at the standard height in ten Saudi regions sourced from NASA [8].
The wind speed changes with the change in height from the surface of the earth, according to Eq. (1), and the Fig. 1 shows this change [9]:
where – mean wind speed at height above ground, – gradient wind speed assumed constant above the boundary layer – height above ground, – nominal height of boundary layer, which depends on the exposure (values for are given in Fig. 1, – power law coefficient).
We note from the observed values of wind speed that the winds are more active in the summer and spring seasons in the northern and central regions, while in the southern regions their average speeds are greater in the second third of the year [10], and this can be seen by looking at Fig. 2.
Fig. 1The wind speed changes with height
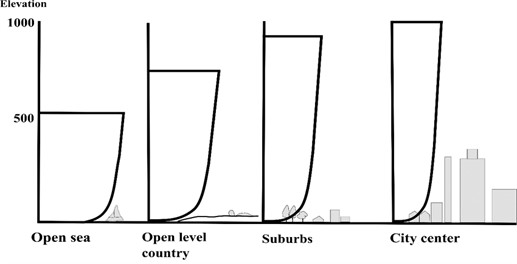
Table 1The mean and max of wind speed (m/s) at gradient height in the ten regions for every month, (NASA)
City month | Wind speed (m/s) | ||||||||||
Riyadh | Makkah | Neom | Dammam | Al Jouf | Red Sea project | Hafar Al-Batin | Jeddah | Asir | Rub’-al Khali | ||
Jan | mean | 2 | 2.4 | 1.6 | 1.9 | 2.2 | 1.5 | 2.1 | 1.9 | 1.2 | 1.4 |
max | 6.3 | 7.4 | 8.1 | 8.3 | 10.7 | 4.9 | 13.8 | 8.5 | 5.6 | 8.2 | |
Feb | mean | 2 | 2.6 | 1.6 | 2 | 2.5 | 1.5 | 2.4 | 2 | 1.3 | 1.5 |
max | 4.6 | 10.2 | 8.6 | 6.6 | 11.7 | 7.4 | 13.5 | 10.3 | 8 | 7 | |
Mar | mean | 2.1 | 2.5 | 1.6 | 2.2 | 2.6 | 1.6 | 2.7 | 1.9 | 1.5 | 1.6 |
max | 5.7 | 9.3 | 7.4 | 5.7 | 14.5 | 7.4 | 16 | 9.2 | 8.5 | 9.2 | |
Apr | mean | 2.1 | 2.1 | 1.6 | 2.3 | 2.8 | 1.7 | 3.1 | 1.8 | 1.6 | 1.7 |
max | 4.9 | 8.2 | 7.1 | 6.9 | 13.8 | 7.1 | 14.9 | 8.1 | 9 | 8 | |
May | mean | 2.1 | 2 | 1.9 | 2.4 | 2.7 | 1.8 | 3 | 1.9 | 1.5 | 1.6 |
max | 7.8 | 7.6 | 7.1 | 9.8 | 13.8 | 7.1 | 14.6 | 8.2 | 6.6 | 7.2 | |
Jun | mean | 2.6 | 2.1 | 1.9 | 2.7 | 2.5 | 1.8 | 3.1 | 2.4 | 1.5 | 1.7 |
max | 7.7 | 8 | 6.8 | 8.7 | 9.9 | 6.8 | 13.2 | 9 | 9 | 8.2 | |
Jul | mean | 2.2 | 2.3 | 2.1 | 2.3 | 2.6 | 2 | 3 | 2.4 | 2.5 | 3.1 |
max | 6.7 | 8.2 | 6.8 | 9.7 | 9.6 | 6.8 | 11.6 | 7.8 | 7 | 11 | |
Aug | mean | 2.1 | 2.1 | 2 | 2.2 | 2.3 | 1.9 | 2.5 | 2.5 | 2.2 | 2.5 |
max | 7 | 8.2 | 6.6 | 8 | 8.8 | 6.6 | 9.3 | 7.7 | 5 | 8 | |
Sep | mean | 1.7 | 2.1 | 1.5 | 1.9 | 2 | 1.6 | 2.3 | 1.7 | 1.7 | 2.1 |
max | 7.1 | 9.9 | 5.2 | 9.1 | 8.8 | 5.2 | 9.9 | 7.7 | 8 | 8.2 | |
Oct | mean | 1.6 | 2 | 1.4 | 1.8 | 2.1 | 1.3 | 1.9 | 1.8 | 1.6 | 1.7 |
max | 6.7 | 8 | 4.3 | 9.7 | 9.3 | 4.3 | 22.1 | 4.8 | 5.3 | 11.1 | |
Nov | mean | 1.8 | 2 | 1.2 | 1.9 | 2 | 1.2 | 1.8 | 1.5 | 1.2 | 1.5 |
max | 5.4 | 6 | 4.1 | 5.8 | 11 | 4.1 | 12.1 | 5.2 | 6 | 12 | |
Dec | mean | 2.1 | 2 | 1.3 | 2.2 | 2 | 1.3 | 1.7 | 1.4 | 1.3 | 1.5 |
max | 6.7 | 6 | 3.5 | 8.7 | 12.1 | 3.5 | 13.2 | 8.1 | 5.2 | 8.2 |
Fig. 2The average annual wind speed in different cities in KSA
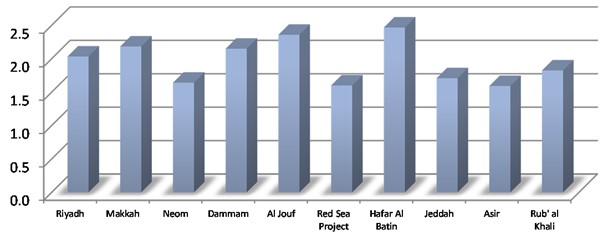
2.2. Renewable energy in Saudi Arabia and vision 2030
The National Renewable Energy Program was launched in the Kingdom of Saudi Arabia, which is a strategic initiative under the umbrella of Vision 2030 and the National Transformation Program [11]. The program is aimed at the sustainable increase in the resurrection of the resources of energy sources in the kingdom to reach 45.3 GW in 2020 AD, that is, equivalent to 4 % of the total production of the Kingdom and 3.27 GW by the year 2023 AD, that is, more than 10 % of the property The energy sector also aspires to be one of the main generators of jobs in the country in the next decade, amid promoting the necessary investments by relying on generating 30 % of energy by 2030 [12].
3. Wind turbines
3.1. Helical-Blade vertical-axis wind turbine
In this type of vertical wind turbine, the average output power is 100 watts when the average wind speed is 9 m/sec, and its operating speed is 3.5 m/sec and with a power factor of 0.15 [13], the Fig. 4 shows the power produced from this turbine when operating in a number of From the cities, by taking the average wind speed in these cities after ignoring the speeds that are less than 3.5 m/s [5].
Fig. 3Helical-blade vertical-axis wind turbine [2]
![Helical-blade vertical-axis wind turbine [2]](https://static-01.extrica.com/articles/22740/22740-img3.jpg)
The electric capacity produced at several heights was calculated in the ten regions, and Fig. 4 the power values in the months of the year and in all the regions under study.
Fig. 4The electrical power at different heights using Helical-Blade vertical-axis wind turbine
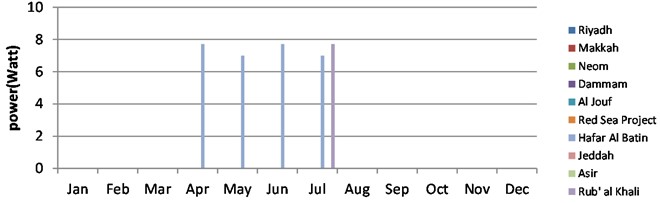
a) 10 m
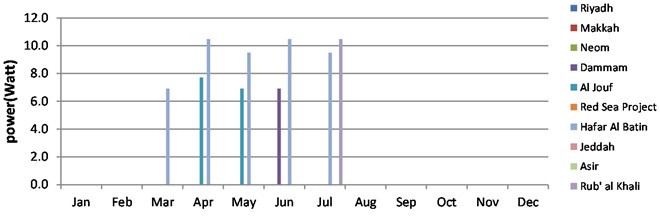
b) 20 m
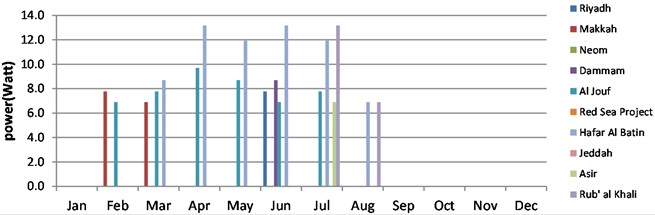
c) 30 m
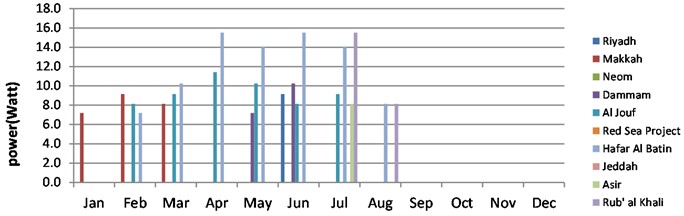
d) 40 m
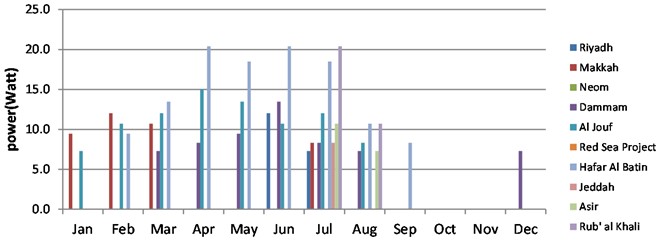
e) 50 m
3.2. Small H-Type Darrieus vertical-axis wind turbine
Energy calculations were made on this type in the ten cities by taking the minimum wind speed required to operate the turbine, and the Table 2 shows the characteristics of this turbine and the following graphs show the electric energy produced at different heights [14].
Fig. 5Small H-Type Darrieus vertical-axis wind turbine
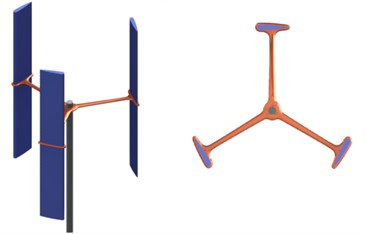
The characteristics of this turbine, are good for employment in small projects. It has a diameter that does not exceed one meter, and the length of the plates is 1.5 meters, and the operating speed is 2 m/s, and this speed is excellent compared to the rest of the small turbines, but what is wrong with this turbine is that the cutting speed is up to 12 m/s. This is a rather low speed, especially if we know that a number of regions have winds that reach much higher than this number for limited periods [15].
When operating this turbine in the areas concerned with the study, it was found that the electric power produced ranged between 8 watts at a height of 10 meters and 22 watts at a height of 50 meters [17], and these values are the maximum values in the different regions, as shown in Fig. 6.
Fig. 6The electrical power at different heights using Small H-Type Darrieus vertical-axis wind turbine
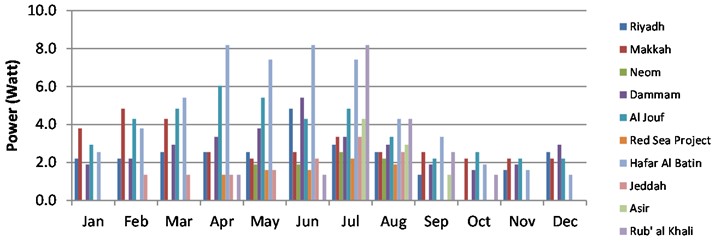
a) 10 m
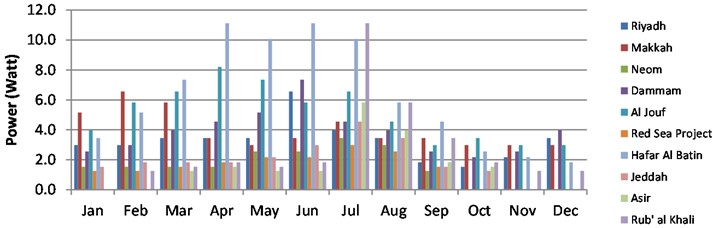
b) 20 m
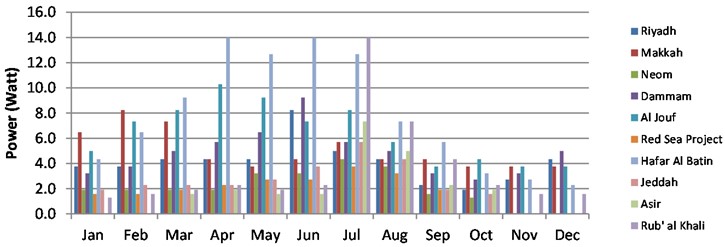
c) 30 m
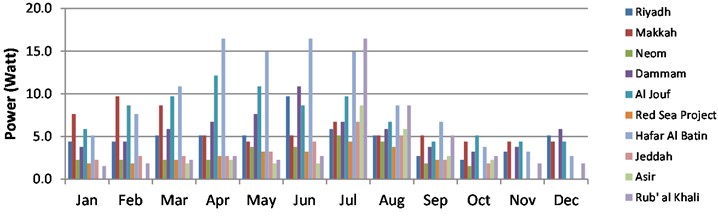
d) 40 m
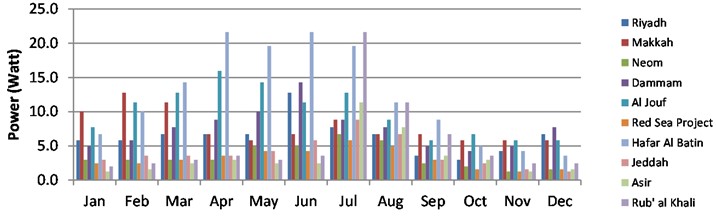
e) 50 m
Table 2Small H-type vertical axes wind turbine parameters [16]
Component | Parameter | Value |
Rotor | Diameter | 1 m |
Length | 1.5 m | |
Weight | 15 kg | |
No. of blades | 3 | |
Materials | Expanded Polypropylene | |
Cut-in wind speed | 2 m/s | |
Rated wind speed | 9 m/s | |
Cut-out wind speed | 12 m/s | |
Generator | Type | Permanent Management 3-Phase AC Generator |
Rated power | 33 W | |
Rotor speed range | 0-400 RPM | |
Pole number | 10 | |
Weight | 18 kg |
3.3. Savonius wind turbine
By taking a plate diameter of 60 cm and a height of 100 cm, the following graphs show the electrical energy produced at several heights.
Fig. 7Savonius wind turbine
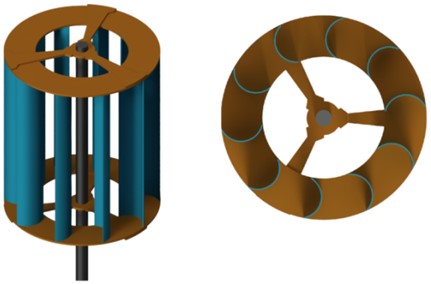
The electrical energy produced when operating this turbine at altitudes of 10 m to 50 m ranges from 12 W to 30 W in the highest wind speed regions as shown in Fig. 8.
Fig. 8The electrical power at different heights using Savonius wind turbine
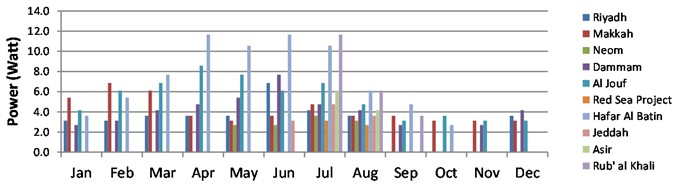
a) 10 m
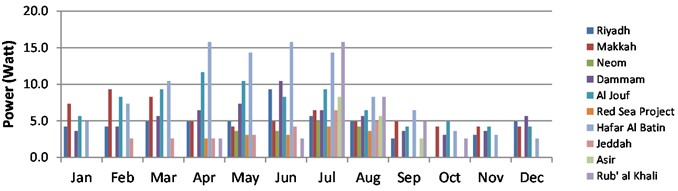
b) 20 m
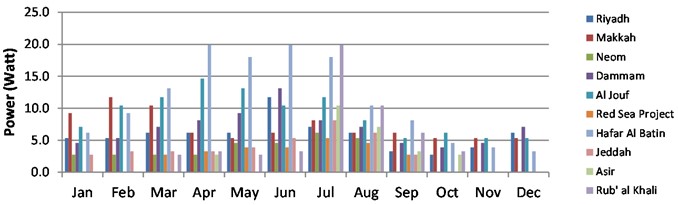
c) 30 m
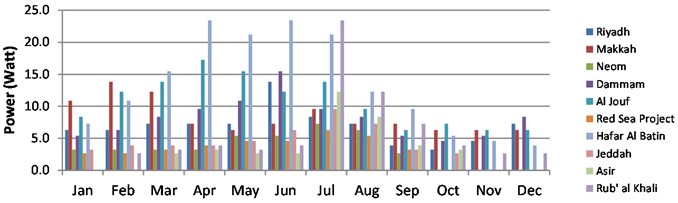
d) 40 m
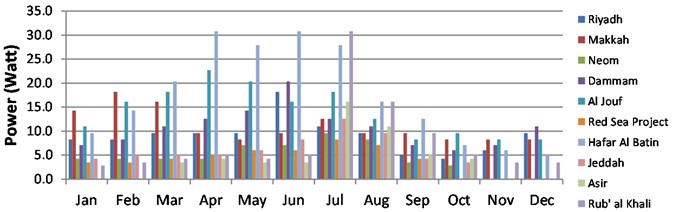
e) 50 m
4. Vertical wind turbine 10 kw
In this turbine, the electric power produced ranged from 8 watts to 22 watts, and this turbine is of the medium type that can be used as a supplier for some small stations or rural homes.
Fig. 9Vertical wind turbine 100 kw
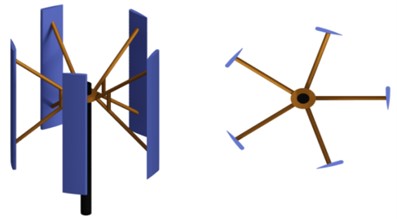
Fig. 10The electrical power at different heights using vertical wind turbine 100 kw turbine
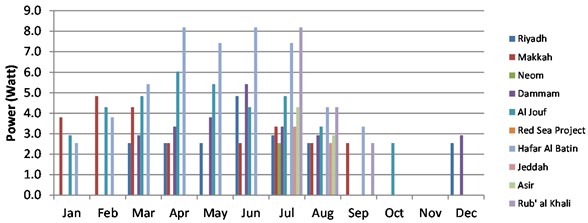
a) 10 m
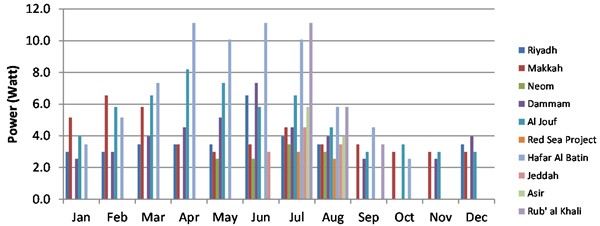
b) 20 m
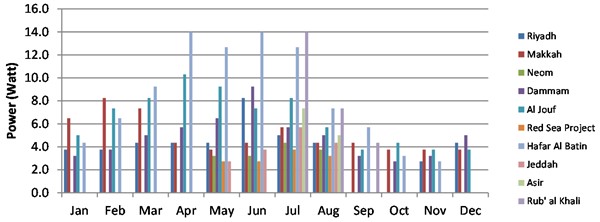
c) 30 m
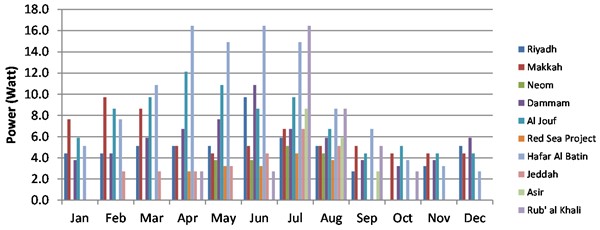
d) 40 m
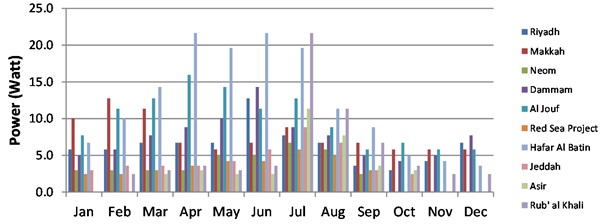
e) 50 m
Table 3Vertical wind turbine 10 kw parameters [18]
Rated power | 10 kW |
Maximum output power | 12 kW |
Output voltage | 300/380 V |
Rotor height | 6.0 m (19.7 ft) |
Rotor diameter | 5.5 m (18.0 ft) |
Start-up wind speed | 2.5 m/s (5.6 mph) |
Rated wind speed | 11 m/s (24.6 mph) |
Survival wind speed | 52.5 m/s (117.4 mph) |
Generator | Permanent magnetic generator |
Generator efficiency | >0.96 |
Turbine weight | 680 kg (1499.1 lbs) |
Noise | <45dB(A) |
Temperature range | –20 °C to +50 °C |
Design lifetime | 20 years |
Fig. 11Average annual GHI in different sites in Saudi Arabia
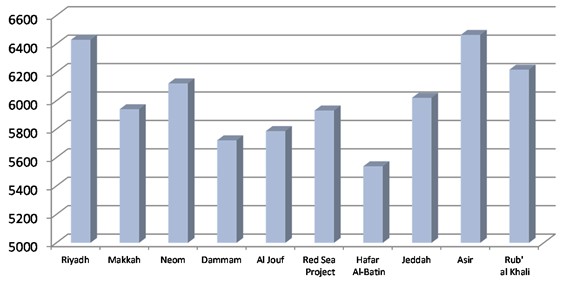
5. Solar energy
The values of radiation (GHI) rates for solar energy in all of the target areas in the study were taken from NASA website, as shown in the Table 4. The GHI values were calculated using Eq. (2):
where: GHI – Global horizontal irradiance, DNI – Direct normal irradiance, DHI – Diffuse horizontal irradiance, SZA – the solar zenith angle.
Table 4GHI for several cities in KSA Over the year (Wh/m2/Day) [19]
City month | Riyadh | Makkah | Neom | Dammam | Al Jouf | Red Sea Project | Hafar Al-Batin | Jeddah | Asir | Rub’al Khali |
Jan | 4270 | 4566 | 4370 | 3570 | 3390 | 4240 | 3230 | 4356 | 5295 | 4710 |
Feb | 5310 | 5288 | 5330 | 4490 | 4440 | 5100 | 4230 | 5247 | 5831 | 5310 |
Mar | 6510 | 6144 | 6210 | 5290 | 5560 | 6030 | 5230 | 6254 | 6711 | 6080 |
Apr | 7430 | 6544 | 7530 | 6150 | 6780 | 6780 | 6070 | 6940 | 7190 | 6840 |
May | 7870 | 6702 | 7970 | 7170 | 7390 | 7140 | 7180 | 7325 | 7224 | 7620 |
Jun | 8580 | 7214 | 8234 | 8000 | 8360 | 7780 | 7940 | 7458 | 7262 | 7630 |
Jul | 8320 | 6947 | 8245 | 7570 | 8150 | 7440 | 7850 | 7640 | 7073 | 7040 |
Aug | 7770 | 6652 | 7325 | 7210 | 7570 | 6940 | 7340 | 7180 | 6921 | 6950 |
Sep | 6930 | 6259 | 5458 | 6530 | 6460 | 6250 | 6330 | 6340 | 6871 | 6680 |
Oct | 5570 | 5761 | 4625 | 5400 | 4760 | 5220 | 4910 | 5230 | 6494 | 6060 |
Nov | 4590 | 4866 | 4215 | 4030 | 3550 | 4350 | 3390 | 4360 | 5577 | 5250 |
Dec | 4000 | 4346 | 3956 | 3250 | 3030 | 3910 | 2770 | 3920 | 5114 | 4460 |
Average | 6429 | 5941 | 6122 | 5722 | 5787 | 5932 | 5539 | 6021 | 6464 | 6219 |
Table 5Electricity produced by solar panels of all types listed in all regions [1]
Solar plate | City | |||||||||
Riyadh | Makkah | Neum | Dammam | Al –Jouf | Red Sea Project | Hafar Al-Batin | Jeddah | Asir | Rub’ al Khali | |
PERC | 1607 | 1485 | 1531 | 1430 | 1447 | 1483 | 1385 | 1507 | 1616 | 1555 |
Monocrystalline | 1286 | 1188 | 1224 | 1144 | 1157 | 1186 | 1108 | 1206 | 1293 | 1244 |
Polycrystalline | 1029 | 951 | 980 | 915 | 926 | 949 | 886 | 965 | 1034 | 995 |
CIGS | 900 | 832 | 857 | 801 | 810 | 830 | 775 | 844 | 905 | 871 |
CdTe | 643 | 594 | 612 | 572 | 579 | 593 | 554 | 603 | 646 | 622 |
Amorphous silicon (a-Si) | 450 | 416 | 429 | 401 | 405 | 415 | 388 | 422 | 452 | 435 |
Fig. 12Electricity produced by solar panels of all types listed in all regions 100 kw turbine
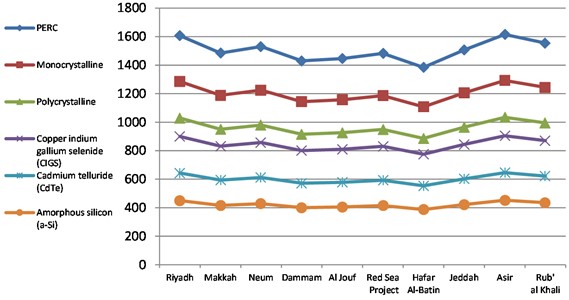
6. Results and discussions
We note that the first turbine starts working at a wind speed of 3.6 m/s, and this is a high speed that makes the application of this turbine in selected areas, of limited benefit at altitudes less than 30 m, but it is possible to apply it at a height of 50 m and more, which limits the possibility of practical applications to use this turbine, while we find that the second turbine operates at wind speeds of 2 m/s, and above and produces electrical energy from 2 watts to 18 watts at a rate of 5.2 watts, and increases its energy production in the summer and spring seasons [6]. As for the third type, it produces energies ranging from 12 watts at a height of 10 m and 30 watts at a height of 50 m, and with a slightly higher efficiency than the second turbine, the fourth turbine.
As for solar energy, the study shows that the first type of panels gives higher energies than the rest of the types, and therefore where one square meter of these panels produces between 1.4 watts, as in the city of Makkah, for example, to 1.8 kilo watts in Asir, compared to the rest of the panels. especially good that some panels give less than 0.5 watts.
The performance of the Helical-Blade turbine was very poor at normal altitudes in all the areas under study, and at higher altitudes, its performance was somewhat acceptable at a height of 50 m in the city of Hafr Al-Batin, but in the rest of the cities it is not convincing, and we also note that it does not work in a monthly 10 and 11 in all regions [20].
As for the rest of the turbines, the energy produced from them was acceptable, reaching 30 watts in some cities, as is the case for the Savonius wind turbine at a height of 50 m.
As for solar energy, we find that GHI rates are good in most areas. The energy values produced range between 400 watts and 1600 watts using different types of solar panels [21].
7. Conclusions
This study shows, that the use of the second type of wind turbine with the use of two solar panels of the first type can provide energy of up to 5 kilowatts at high altitudes (30 m and higher) sufficient to operate a communications tower, for example, and up to 2 kilowatts at a height 10 m and enough to operate lights and chargers for stretchers in the bus parking lot.
It is also not recommended to use the Helical-Blade turbine in different areas and at lower altitudes, and the Vertical wind turbine 10 kw can be used depending on the good results that have been achieved for this turbine.
In Hafr Al-Batin, most turbines can be used due to good wind speed rates, and in cities such as Riyadh and Asir, we find that solar energy is high, so it can be relied upon in many small projects, such as street lighting, for example.
References
-
A. Suryadi, P. T. Asmoro, and A. Solihin, “Hybrid electric power plant using wind turbine savonius helix and solar cell as an alternative power source in the lightning tower at flashing lights,” ADI Journal on Recent Innovation (AJRI), Vol. 1, No. 1, pp. 1–6, Sep. 2019, https://doi.org/10.34306/ajri.v1i1.93
-
E. Möllerström, P. Gipe, J. Beurskens, and F. Ottermo, “A historical review of vertical axis wind turbines rated 100 kW and above,” Renewable and Sustainable Energy Reviews, Vol. 105, pp. 1–13, May 2019, https://doi.org/10.1016/j.rser.2018.12.022
-
K. S. Alqdah, R. Alahmdi, A. Alansari, A. Almoghamisi, M. Abualkhair, and M. Awais, “Potential of wind energy in Medina, Saudi Arabia based on Weibull distribution parameters,” Wind Engineering, Vol. 45, No. 6, pp. 1652–1661, Dec. 2021, https://doi.org/10.1177/0309524x211027356
-
B. Zohuri, Hybrid Energy Systems: Driving reliable renewable sources of energy storage. Cham: Springer International Publishing, 2018, https://doi.org/10.1007/978-3-319-70721-1
-
S. A. Kalogirou, “Wind energy systems,” Solar Energy Engineering, Vol. 105, No. 11, pp. 735–762, 2014, https://doi.org/10.1016/b978-0-12-397270-5.00013-3
-
W. Tjiu, T. Marnoto, S. Mat, M. H. Ruslan, and K. Sopian, “Darrieus vertical axis wind turbine for power generation I: Assessment of Darrieus VAWT configurations,” Renewable Energy, Vol. 75, pp. 50–67, Mar. 2015, https://doi.org/10.1016/j.renene.2014.09.038
-
A. Rezaeiha, H. Montazeri, and B. Blocken, “On the accuracy of turbulence models for CFD simulations of vertical axis wind turbines,” Energy, Vol. 180, pp. 838–857, Aug. 2019, https://doi.org/10.1016/j.energy.2019.05.053
-
D. Han, Y. Heo, N. Choi, S. Nam, K. Choi, and K. Kim, “Design, fabrication, and performance test of a 100-W helical-blade vertical-axis wind turbine at low tip-speed ratio,” Energies, Vol. 11, No. 6, p. 1517, Jun. 2018, https://doi.org/10.3390/en11061517
-
K. Almutairi, S. S. Hosseini Dehshiri, S. J. Hosseini Dehshiri, A. Mostafaeipour, A. Issakhov, and K. Techato, “Use of a hybrid wind-solar-diesel-battery energy system to power buildings in remote areas: A case study,” Sustainability, Vol. 13, No. 16, p. 8764, Aug. 2021, https://doi.org/10.3390/su13168764
-
M. Parol, B. Arendarski, and R. Parol, “Calculating electric power and energy generated in small wind turbine-generator sets in very short-term horizon,” E3S Web of Conferences, Vol. 84, p. 01006, 2019, https://doi.org/10.1051/e3sconf/20198401006
-
P. Jenkins, M. Elmnifi, A. Younis, and A. Emhamed, “Hybrid power generation by using solar and wind energy: case study,” World Journal of Mechanics, Vol. 9, No. 4, pp. 81–93, 2019, https://doi.org/10.4236/wjm.2019.94006
-
J. Khan and M. H. Arsalan, “Solar power technologies for sustainable electricity generation – A review,” Renewable and Sustainable Energy Reviews, Vol. 55, pp. 414–425, Mar. 2016, https://doi.org/10.1016/j.rser.2015.10.135
-
N. Cajethan, U. C. Uchenna, and M. Theophilus, “Wind-solar hybrid power system for rural applications in the South Eastern states of Nigeria,” Journal of Electrical Systems, Vol. 8, No. 3, pp. 304–316, 2016.
-
M. B. Ageze, “Design and manufacturing of direct driven standalone wind energy conversion system,” MSc Thesis, 2013, https://doi.org/10.13140/rg.2.1.1031.4404
-
E. Zell et al., “Assessment of solar radiation resources in Saudi Arabia,” Solar Energy, Vol. 119, pp. 422–438, Sep. 2015, https://doi.org/10.1016/j.solener.2015.06.031
-
C. Azorin-Molina et al., “Recent trends in wind speed across Saudi Arabia, 1978-2013: a break in the stilling,” International Journal of Climatology, Vol. 38, pp. e966–e984, Apr. 2018, https://doi.org/10.1002/joc.5423
-
A. Aghahosseini, D. Bogdanov, L. S. N. S. Barbosa, and C. Breyer, “Analysing the feasibility of powering the Americas with renewable energy and inter-regional grid interconnections by 2030,” Renewable and Sustainable Energy Reviews, Vol. 105, pp. 187–205, May 2019, https://doi.org/10.1016/j.rser.2019.01.046
-
U. Divakaran, A. Ramesh, A. Mohammad, and R. K. Velamati, “Effect of helix angle on the performance of helical vertical axis wind turbine,” Energies, Vol. 14, No. 2, p. 393, Jan. 2021, https://doi.org/10.3390/en14020393
-
S. C. Johnson, D. J. Papageorgiou, D. S. Mallapragada, T. A. Deetjen, J. D. Rhodes, and M. E. Webber, “Evaluating rotational inertia as a component of grid reliability with high penetrations of variable renewable energy,” Energy, Vol. 180, pp. 258–271, Aug. 2019, https://doi.org/10.1016/j.energy.2019.04.216
-
R. M. Moore et al., “Extensive hydrogen supersaturations in the western South Atlantic Ocean suggest substantial underestimation of nitrogen fixation,” Journal of Geophysical Research: Oceans, Vol. 119, No. 7, pp. 4340–4350, Jul. 2014, https://doi.org/10.1002/2014jc010017