Abstract
we can obtain porous netshaped conformation after activate treatment, such conformation will make area of the sample surface increase, surface energy increase. Reticular conformation and nanometer hole structure increase the combination intensity with the bone. As implanted material, for human bone tissue through the mesh-like surface of the conditions of the exchange offer. Therefore, bionic activation layer/titanium biomedical materials may become the optimization implant materials for its excellent biological properties. In the course of simulated body fluid (SBF) cultivation, Titanate gel layer in simulated body fluid absorpt calcium ion, hydroxyapatite in titanate layer of porous network structure form nuclear and grow up and induced deposition a certain orientation of the bone-like apatite.
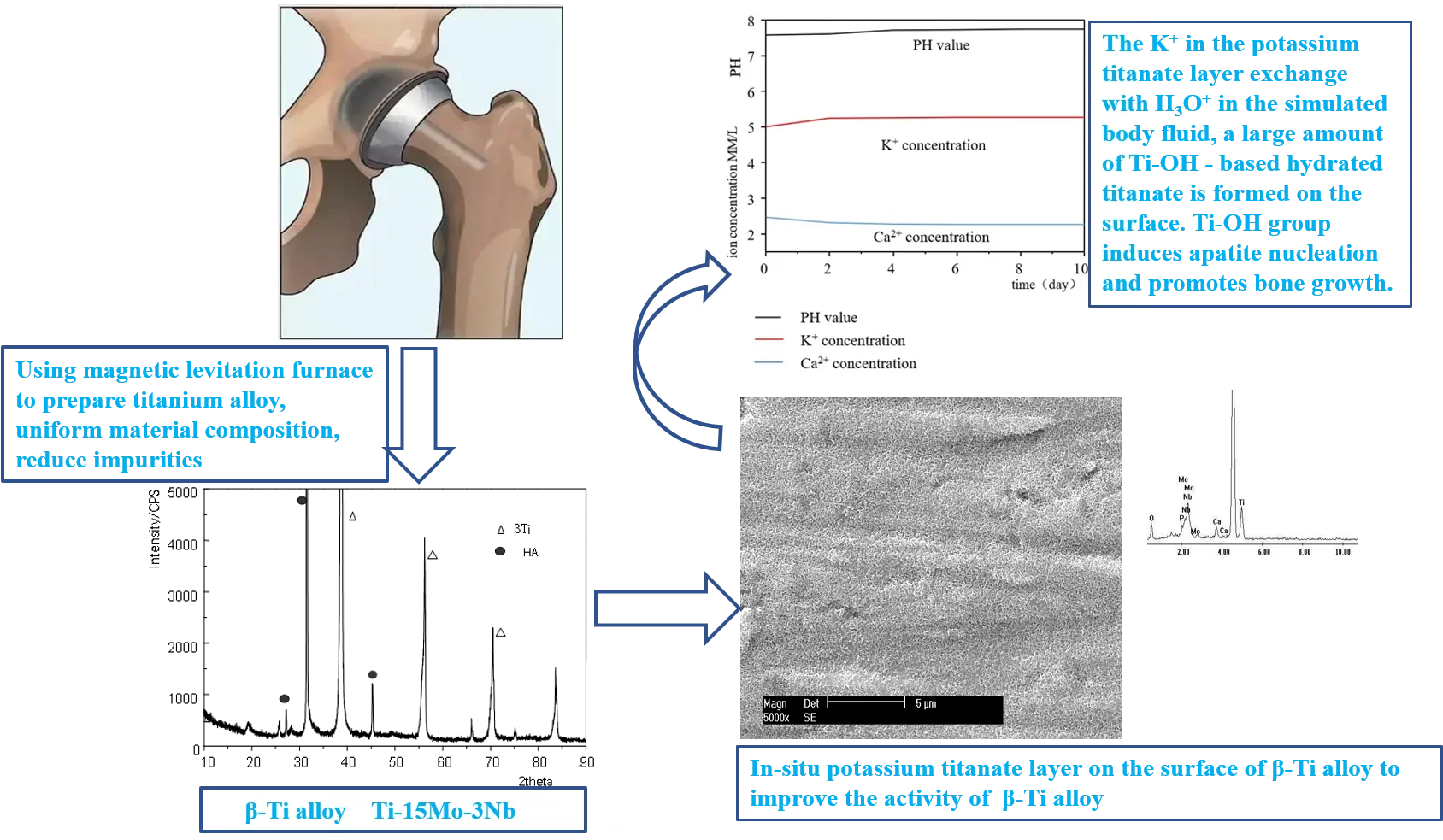
Highlights
- Using magnetic levitation furnace to prepare titanium alloy, uniform material composition, reduce impurities.
- In order to improve the activity of titanium alloy, the titanium alloy needs to be activated. Titanium alloy was embedded with potassium titanate powder and heated at high temperature in a box-type resistance furnace. Potassium titanate layer was generated in situ on the surface of titanium alloy and formed a strong chemical bond with the titanium alloy matrix.
- SBF solution culture was used to test the biocompatibility of titanium alloy. The K+ in the potassium titanate layer exchange with H3O+ in the simulated body fluid, a large amount of Ti-OH - based hydrated titanate is formed on the surface. Ti-OH group induces apatite nucleation and promotes bone growth.
1. Introduction
Titanium and titanium alloys, as hard tissue repair and replacement materials, have been widely applied and valued in clinic for their excellent biocompatibility, mechanical properties and corrosion resistance. However, since the surface of the implant is still bioinert, it is hoped that the biocompatibility and bioactivity of the implant can be further enhanced by surface modification, so that the implant can form a solid biological bond with the bone tissue. At present, plasma spraying is the most common method to obtain hydroxyapatite coating, which has been applied in clinical practice. However, the coating obtained by this method has unstable characteristics due to rapid curing, uneven composition, easy degradation, and is very easy to separate from the surface and be absorbed by the human body [1]. As a result, implant implantation failed, so people began to try to enhance the biological activity of titanium alloy surface by other surface modification methods [2].
In order to improve the activity of titanium alloy, the activation treatment was carried out to generate in-situ potassium titanate layer on the surface of titanium alloy and form a strong chemical bond with the titanium alloy matrix. The activated porous network structure increases the surface area and the surface energy is relatively high. The mesh structure and nanoscale pore structure increase the binding strength to bone. When used as implant material, it provides conditions for the exchange of human bone tissue through the mesh surface. Therefore, biomimetic activated layer/titanium alloy biomedical materials are expected to be implanted materials with excellent biological properties.
2. Experimental
Self – made low modulus β titanium alloy was used as the base. The samples were polished with 280 #, 400 # and 600 # sandpaper successively, and cleaned with acetone and deionized water by ultrasonic wave. The surface of the sample was activated and dried at 40 ℃ for 24 hours.
The samples to be cultured were washed with deionized water and dried in a drying oven at 37 ℃, then immersed in simulated body fluid (SBF) at pH 7.4 for culture, with 30 mL simulated body fluid for each sample. The beaker was sealed with plastic wrap and placed in a drying oven at 37 ℃. The simulated body fluids were replaced every two days. The concentration changes of K+ and Ca2+ ions in simulated body fluids were measured by electrolyte analyzer at a certain interval. After a certain number of days of culture, the samples were taken out and cleaned by ultrasonic with anhydrous ethanol as medium, washed by deionized water and dried. Then, the morphology of calcium and phosphorus layer on the surface was observed under scanning electron microscope and energy spectrum analysis was performed.
3. Results
3.1. Surface morphology and surface element analysis of sedimentary layer
The surface morphology of gradient coating material formed by SBF immersion was observed under scanning electron microscope to understand the microstructure characteristics of the material. The results after soaking for 1 week are shown in Fig. 1. The surfaces are completely covered by an accumulation layer, and calcium phosphate deposits begin to form into balls. At high magnification, there are a large number of micropores, as shown in Fig. 1(b). It can be seen that the surface of sample soaked in a short time has the characteristics of needle network.
Fig. 1Surface morphology of samples cultured in SBF for 1 week
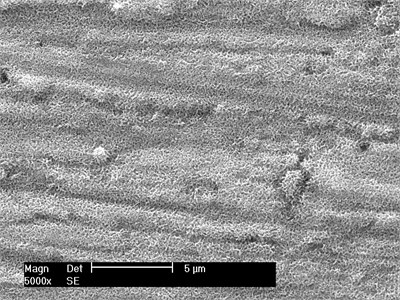
a) Low magnification
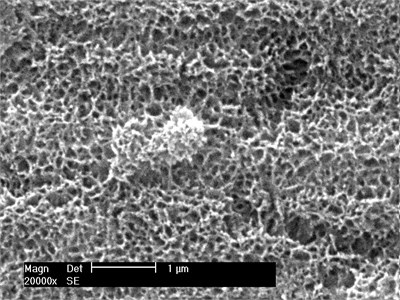
b) High magnification
Fig. 2Surface morphology of samples cultured in SBF for 2 weeks
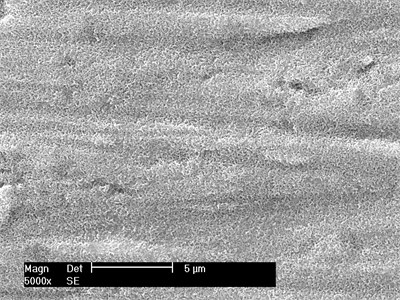
a) 3 days
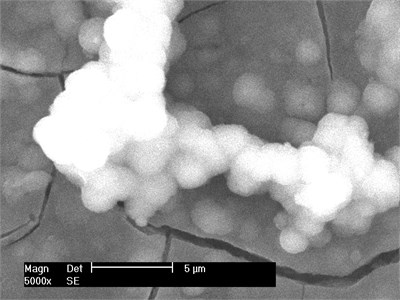
b) 2 weeks
The surface morphology was observed after soaking in SBF for different time. After 3 days of culture in SBF, the sediment layer on the surface of the sample was very thin, and the calcium phosphate had not been deposited into a ball under scanning electron microscopy, as shown in Fig. 2(a). After two weeks of culture in SBF, the sediment layer on the sample surface was significantly thickened, as shown in Fig. 2(b). On the sample cultured for 2 weeks, a thick layer of calcium phosphate was deposited on the porous network structure formed by surface modification, and many small spherical layers of calcium phosphate were formed on the surface. The average pellet size is about 2 microns. The pellets grow with immersion time. Each pellet absorbs Ca from SBF, and P ions gradually grow to cover the entire porous surface of the sample.
The calcium phosphate layer on the surface of the sample is uniform and smooth as a whole, and there are small cracks locally. The reason for the interlinked microcracks may be that there are more water molecules in the phosphate deposition layer induced in the liquid phase. When the sample is taken out of the simulated body fluid and dried, the water molecules evaporate, and the microcracks appear on the surface of the sediment layer due to dehydration and contraction.
The results of EDS analysis showed that the particles formed in SBF were calcium phosphate rich in Ca and P. Fig. 3 shows the energy spectrum of titanium alloy samples treated with acid and alkali after 1-week simulated body fluid culture. As can be seen from the figure, in addition to matrix elements, the main elements in EDS spectrum are calcium, phosphorus and oxygen. According to the surface morphology, a continuous apatite layer formed by the accumulation of spherical apatite particles has been formed on the surface of the sample after soaking for a week. With the extension of soaking time, the apatite layer gradually thickens.
Fig. 3Energy spectrum after simulated body fluid culture for 1 week
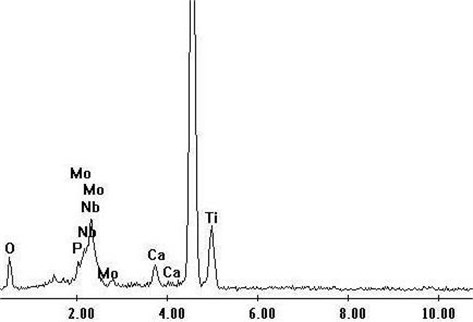
3.2. Phase analysis of calcium and phosphorus deposits
Fig. 4 shows the XRD pattern of the surface of the sample treated with acid-base activation soaked in simulated body fluid for a certain time, and its main phase structure is HA. It can be seen from the XRD patterns of the samples soaked for different time that the intensity of the diffraction peak of apatite gradually increases with the increase of soaking time. As shown in Fig. 3, after soaking for two weeks, the diffraction peak of hydroxyapatite obviously appeared in the atlas, and the diffraction peak of β -type titanium alloy on the original substrate still existed, proving that the surface hydroxyapatite layer was relatively thin. The characteristic peaks (211), (112) and (300) combined to form a broad peak, indicating that the generated apatite layer has a low degree of crystallization and may contain some amorphous materials. Therefore, this apatite is called osteoid apatite, that is, like bone apatite, is weakly crystallized apatite.
By using biomimetic growth in simulated body fluid generated hydroxyl apatite, its composition, phase structure and similar inorganic qualitative structure of the human body tissue, have lower crystallinity, from a biological point of view, apatite structure of incomplete is helpful to get a better biological activity, so this kind of apatite with good biocompatibility.
Fig. 4Energy spectrum after simulated body fluid culture for 2 weeks
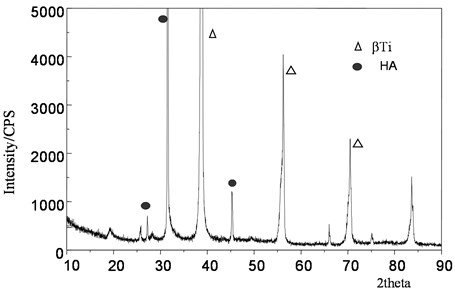
3.3. Analysis of simulated ionic concentration changes in body fluids
The samples treated by activation process were immersed in simulated body fluid for different time, and the concentrations of potassium ion and calcium ion in SBF liquid were measured. The variation trend of ion concentration obtained is shown in Fig. 5. It can be found that the concentration of potassium ions gradually increases, while the concentration of calcium ions gradually decreases, decreasing rapidly in the first two days, and then tends to moderate.
Fig. 5Curve of potassium ion and calcium ion concentration in SBF liquid
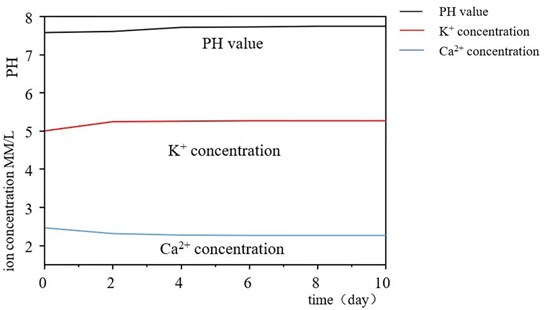
The increase of potassium ion concentration is due to the dissolution of potassium ion from the potassium titanate layer on the surface of the sample in simulated body fluid, while the decrease of Ca and P ion concentration is due to the formation of apatite on the surface of the sample, and the consumption of Ca and P ion with its growth. The K ions in the potassium titanate layer exchange with H3O+ in the simulated body fluid, forming a large amount of Ti-OH hydrated titanate on the surface of the sample when the pH value increases. This Ti-OH group induces the nucleation of apatite, whose crystal nuclei grow by absorbing Ca and P ions from the surrounding simulated body fluids.
4. Discussion
After activation treatment, the sample surface presents fine mesh like micro-cracks, which increases the surface area and is electronegative [3]. It is conducive to sequential adsorption of Ca2+ and PO43- ions, and provides more nucleation points for apatite deposition, so that the coating and the substrate bite and combine to form a firm chemical bond, and improve the bonding strength of the coating. In addition, TiO2 generated on the surface of the sample due to oxidation will react with KOH, forming a layer of Ti-OH hydrogel on the surface of the sample to adsorb bioactive groups or functional groups, such as COOH, OH, NH2 and CO. When The Ti-OH gel is partially ionized, Ca2+ ions in the solution are absorbed by static electricity and then combined with PO43- ions to form a strong chemical bond [4]. After a period of time, the concentration of Ca2+ and PO43- ions in the local mesh on the surface of the sample reached a certain supersaturation and began to nucleate and grow [5].
In simulated body fluids, calcium and phosphorus were induced to form a new phase core through electrostatic action on the surface of microporous titania [6]. Since the surface of titanium alloy is a microporous structure with submicron structure, and the surface composition is mainly TiO(OH)2 and K2TiO3, the surface is rich in negative charge (OH-) from the point of view of charge, which meets the basic requirements of biological mineralization. In the concave part of the material, the charge is more concentrated, which is more conducive to attracting ions that can form crystal nuclei through electrostatic attraction, thus forming crystal nuclei [7]. Then phosphate and calcium ions co-deposition, apatite heterogeneous nucleation and crystallization growth, the formation of osteoid apatite.
The main process of surface liquid phase deposition of bioceramic coating can be summarized as follows: (1) activation of titanium alloy surface, formation of Ca2+ and PO43- ion adsorption nucleation points; (2) Adsorbed and nucleated at the local activation points on the surface to form a new spot-like HA phase; (3) Under the conditions of thermodynamics and kinetics, the new phase gradually grows up to form spherical HA phase; (4) The HA phase expands and thickens to form a network structure. (5) Finally, THE HA layer is spread over the whole surface, and the visible white coating appears macroscopically, and the needle shape appears microscopically.
5. Conclusions
The above studies show that the porous network and weakly crystalline titanate gel layer are formed on the surface of the alloy after activation treatment. In the process of simulated body fluid culture, titanate gel layer absorbs calcium and phosphorus ions from simulated body fluid, and hydroxyapatite [Ca10(PO4)6(OH)2] nucleates and grows on the porous network structure of titanate gel layer. The deposition of osteoid apatite with certain orientation is induced, which indicates that titanate gel layer has good biological activity.
References
-
Ning Congqin, “Development and research status of medical titanium alloy,” Materials Science and Technology, Vol. 10, No. 1, pp. 100–106, 2002.
-
Zeng Shengyu, “Advances in surface modification of metallic biomaterials,” Materials Protection, Vol. 33, No. 1, pp. 5–8, 2000.
-
Kim Hm, Miyaji F., Kokubo T., and Nakamura T., “Effect of heat treatment on apatite-forming ability induced by alkali treatment,” Journal of Materials Science: Materials in Medicine, Vol. 7, No. 1, pp. 341–347, 1997.
-
Yuan Xuemin, “Study on surface activation of Ti-15Mo-3Nb alloy,” Journal of Hebei University of Technology, Vol. 24, pp. 4–10, 2006.
-
Zhang Yaping, “Composite bioceramic coatings were prepared by one-step excited photocoagulation on titanium alloy surface,” Journal of Materials Research, Vol. 8, No. 3, pp. 4–10, 1998.
-
Ning Congqin, “Microstructure of HA/BG biocomposite coatings on pure titanium,” Materials Science and Technology, Vol. 10, No. 1, pp. 30–33, 2000.
-
Yang Xianjin, “Effect of Calcium and phosphorus layers on the binding properties of NiTi shape memory alloy to bone tissue,” Journal of Materials Heat Treatment, Vol. 23, No. 1, pp. 40–42, 2002.
-
H. B. Wen, J. G. C. Wolke, J. R. de Wijn, Q. Liu, F. Z. Cui, and K. de Groot, “Fast precipitation of calcium phosphate layers on titanium induced by simple chemical treatments,” Biomaterials, Vol. 18, No. 22, pp. 1471–1478, Jan. 1997, https://doi.org/10.1016/s0142-9612(97)82297-1
About this article
Thanks to the guidance of Teacher Cui Chunxiang of Hebei University of Technology and the support of biomedical Materials Laboratory and Testing Center. They provided help during the research and preparation of the manuscript.