Abstract
A reliable operation of generators is highly dependent on the electrical and mechanical integrity of stator end-winding vibration and has been a major concern in the last two decades. This paper focuses on the experience in hammer impact testing of end-winding vibrations of parallel phase leads and series loop caps in two hydro generators in a different temperature state. The measurements were conducted using hammer testing on the two generators separately and a discussion and comparison of the results was presented in order to perceive the dependence of the temperature and dynamic characteristics of the structures. Introductory, a brief survey of recent research progress in monitoring the end-winding vibrations in generators is presented. Subsequently, a detailed description of the performed measurement, starting from the identification of the object and conducted testing methodology according to established standards is systematically exposed. Ultimately, a paramount accent will be put on the experimental results for the measured natural frequencies of the generators, perspectives and propositions on subsequent scientific will definitely not be left out.
1. Introduction
In 2011, the amount of operating electrical machines in the world was around 16 billion with a serious growth tendency of 50 % in the following 6 years [3]. These machines undergo failure processes from vibrational nature due to thermal, electrical, mechanical or environmental stresses which are in details discussed in [1, 2]. A modern and extensive discussion of the new techniques related to condition monitoring and fault diagnosis for electrical machines is given in [3, 4]. One of the most complex parts of a generator to design and fabricate is the end-winding region which makes it most submissive to faults. Long-term end-winding vibrations might cause premature winding failure [5-7]. The dynamic response of stator end-winding bars resulting in vibration can be attributed to the electromagnetic frequency at twice power frequency (100 Hz) and electromagnetic forces between two adjacent bars are proportional to the square of the current [7, 8]. Since the windings and supports largely determine the rigidity, the value of the resonant frequency is dependent on the rigidity of the winding or support structure. During a longer period of time, the insulation and supports can become loose and their natural frequency can become lower [12]. Stator windings that are in resonance will have high levels of vibration, if not properly damped or braced. Evidence of high vibration can sometimes be seen through visual inspection, with observance of dusting and greasing or through experimental, analytical, and numerical methods have been utilized [9]. There are two primary experimental methods to anticipate and detect end winding resonant vibration – the hammer impact testing or also known as “bump test” and on-line monitoring [10, 14]. Details about the required instrumentation and procedure of bump testing are given in [11-13]. Online monitoring method is based on using fiber-optic accelerometers which are profoundly explained in [11, 14]. In section 2 will be briefly introduced a description of the considered problem in the hydro-generator, followed by section 3 where the methodology of the conducted impact hammer testing of end-winding vibrations are explained. At the end concluding remarks and intentions for future work in the field are presented.
2. Problem statement
The considered hydro power plant contains two synchronous hydro-electrical generators with rotating speed of 300 rpm. The first generator has been in a working state shortly before the measurement was implemented and the second one is in a repairing phase for a longer period of time. The main external exciting force of the dynamic response of stator end-winding bars which results in vibration is the electromagnetic frequency at twice power frequency (100 Hz). The natural frequency of each parallel phase lead and series loop cap is controlled by performing an impact hammer testing (Fig. 1).
Fig. 1Measurement points
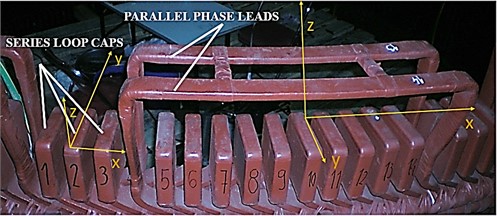
Initially, the outcome of the hammer impact measurement in this case is to check the natural frequencies of the parallel phase leads and series loop caps of the stator in repairing phase in order to detect the ones which are vibrating in the resonant region. Secondly, the same parallel phase leads and series loop caps are tested in the warm generator in order to come to a conclusion for the influence of the temperature on the dynamic characteristics (natural frequency and rigidity) of the generators.
3. Measurement methodology
For these generators an impact hammer testing with an exciter which is not attached to the construction and an impulse function as an excitation according to ISO 7626 was chosen. On both generators were chosen the same end-winding caps and bars. Before the measurement of the natural frequencies, the temperature with an infrared thermometer was determined. The first generator (G1) was in a cold state and the second generator (G2) was in a warm state. Furthermore, the points where the impact with the hammer are implemented and the accelerometer will be placed are defined. The measurement points provided information about the natural frequencies in three directions: tangential (-axis), radial (-axis) and axial (-axis) as shown in the Fig. 2.
Fig. 2Directions of measurement
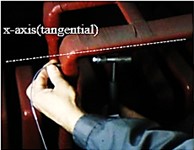
a)
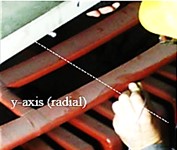
b)
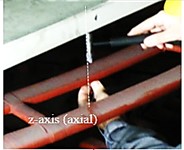
c)
According to ISO 7626:1 and ISO 7626:5, the validity of the signal is defined through the minimal value for the coherence function which is 0,90. The coherence function based on averaging three samples (three hammer “bumps” on a same measurement point and in same direction) provides control over the repeatability of the received values for the natural frequency. The phase spectra have a crucial role during identification of the characteristic natural frequencies in the FRF spectra. The phase will be within 10 % of 90° at a natural frequency. As a stimulus frequency approaches the undamped natural frequency, the magnitude approaches a maximum and a phase shift crosses through 90°.
4. Measurement results
During the measurement, FRF, coherence and phase spectra for every measurement point were provided and saved for later observation. All of the graphs were in details analysed and the natural frequencies for all of the points based on the before mentioned criteria were provided. Given the results of the foregoing experiments, it is noticed that some of the measurements of the bars in tangential and axial direction show an existence of natural frequency which belong in the resonant region of 100 Hz. Furthermore, one interpretation of the results is that a dependence between the temperature and the structural parameters of the generators is noticed. In the radial -direction, the natural frequencies of the bars seem to significantly become higher as the temperature decreases, while in tangential and axial direction it is not drastically changed. Natural frequencies of the caps were measured only in radial direction and similarly, there is a notable change in the natural frequency as the temperature lowers.
Fig. 3Coherence, phase spectra and FRF for the loop cap in y-direction on Generator 1
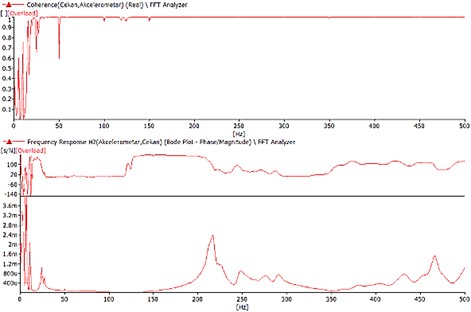
Fig. 4Coherence, phase spectra and FRF for the lop cap in y-direction on Generator 2
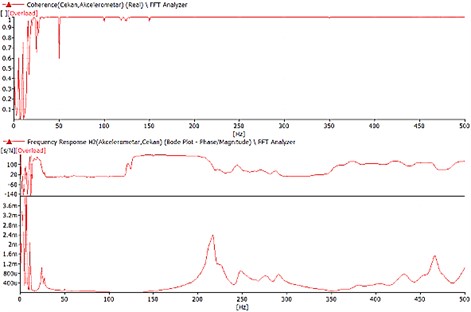
As it can be seen from Fig. 3, the natural frequency of the loop caps belonging to the cold generator in -direction is 251,25 Hz. The natural frequency of the loop caps belonging to the warm generator is 216,25 Hz (Fig. 4) which is significantly lower. Regardless, it is hard to determine the pattern of which the natural frequency changes depending on the temperature variations. As before mentioned, even though theoretically identical, the two generators are still different structures in reality which makes it difficult to be decisive about the relation between the temperature and natural frequency changes.
5. Conclusions
The results suggest that the parallel phase leads and caps that showed a resonant frequency within the resonant frequency region should be replaced. The two generators, even though manufactured as identical, they still behave uniquely with individual natural frequencies. This makes it hard to make a conclusive statement about the dependence of the changes in temperature over the natural frequencies of end-windings even though a difference is noticed. Consequently, the outcome of the results analyses leads to conclusion that the impact hammer testing should be conducted on a single generator during a process of cooling. Future work will be invested in conducting repeatable measurements of a parallel phase lead or a cap in same direction and same measurement point during a process of decreasing the temperature of a single generator. These measurements will provide precise results on how the natural frequency values vary depending on the change of temperature. Ultimately, the relation between these two physical and dynamical parameters will lead to a more aware and accurate monitoring and detecting of the generator windings natural frequency problems and avoiding damages thereof.
References
-
Stone G. C. Condition monitoring and diagnostics of motor and stator windings – a review. IEEE Transactions on Dielectrics and Electrical Insulation, Vol. 20, Issue 6, 2013, p. 2073-2080.
-
Maughan P. E. Monitoring of generator condition and some limitations thereof. Electrical Insulation Conference and Electrical Manufacturing Expo, 2005.
-
Henao H., Capolino Ga, Fernandez Cabanas M., Filippetti F., Bruzzese C., Strangas E., Pusca R., Estima J., Riera Guasp M., Hedayati Kia S. Trends in fault diagnosis for electrical machines: a review of diagnostic techniques. IEEE Industrial Electronics Magazine, Vol. 8, Issue 2, 2014, p. 31-42.
-
Tavner P. J. Review of condition monitoring of rotating electrical machines. IET Electric Power Applications, Vol. 2, Issue 4, 2008, p. 215-47.
-
Lin R., Laiho An, Haavisto A., Arkkio A. End-winding vibrations caused by steady-state magnetic forces in an induction machine. IEEE Transactions on Magnetics, Vol. 46, Issue 7, 2010, p. 2665-2674.
-
Letal J., Warren V., Power Q. I. Optimize stator endwinding vibration monitoring with impact testing. The Premier Electrical Maintenance and Safety Event, 2017.
-
Kapler J., Letal J., Sasic M., Stone G. C., Power Qualitrol I. Recent endwinding vibration problems in air-cooled turbine generators. CIGRE, 2014.
-
Letal, Teixeira M. End-Winding Vibration Monitoring of Turbo-Generators. Iris Power, 2016.
-
Zhao Y., Yan B., Chen C., Deng J., Zhou Q. Parametric study on dynamic characteristics of turbogenerator stator end winding. IEEE Transactions on Energy Conversion, Vol. 29, Issue 1, 2014, p. 129-137.
-
Moore B., Maughan C. Generator stator end winding resonance: problems and solutions. ASME 2013 Power Conference, 2013.
-
Shally D., Farrell M., Sullivan K. Generator end winding vibration monitoring. 43rd International Universities Power Engineering Conference, 2008.
-
Ellis M., Kraijesteijn J. Assessing the integrity of generator stator end windings. 20th International Conference on Nuclear Engineering and the ASME 2012 Power Conference, 2012.
-
Maughan C. V. Vibration detection instrumentation for turbine-generator stator endwindings. Electrical Insulation Conference, 2009.
-
Sasic M., Jiang H., Stone G. C. Requirements for fiber optic sensors for stator endwinding vibration monitoring. International Conference on Condition Monitoring and Diagnosis, 2012.