Abstract
The constructed 3D model of the lower part of human body for the simulation of high jump enables to investigate not only parameters of the jump but to analyses the forces acting in muscles and joints as well. The model enables determining the most important muscles for the jump performance and sequentially to make recommendations for the sportsmen seeking the jump height. The developed methodology can be applied for the analysis of other type movements in sports.
1. Introduction
The physical ability to spring is important for the majority of sportsmen (such as those involved in basketball, volleyball, or tennis). Various sources describe spring a little differently. The capability of jumping means that a person is able to jump far or high. Springing is the ability to raise oneself higher or further with the help of the take-off force. Jumping is one of the most important aspects of a sportsman’s complex ability, which depends on the speed of contraction of leg muscles and on the coordination of movements (arm swing). Springing is the human and animal ability to raise the body off a support for a short period of time using only their own muscle strength. It is obvious from the given definitions that ‘spring’ may be defined as the ability to jump high and fast. Spring may be tested in various ways: by performing various jumps up from an inert position (with squatting, from a squat as the initial position, off a platform, with arm swing, without arm swing etc.) and by registering the vertical component of the ground support’s reaction or by measuring the increase of body mass center (height of jump) by other methods. Usually a sportsman is given the task to jump a maximum height (high jump). Testing of spring by estimating jump height is the most common method. Very rarely a sportsman who is being examined is requested to jump as high and as fast as he can (fast jump) [1].
Motion analysis methods are applied in human gait analysis and the analysis of movement characteristics for different branches of sports. Human movements can be recorded and analyzed even by a single camera simply recording the analyzed subject and later reconstructing the recorded view in a slowed-down manner. However, 3D motion analysis systems [1] (such as Vicon, Qualisys, Simi, Ariel and others) usually consist of 1-8 stationary and mobile high velocity digital cameras, a device for information transfer from cameras to computer and special software (such as Visual 3D or Simi among others) for the acquisition of measurement information on motion parameters. For more comprehensive analysis of the mentioned systems, synchronous operating force platforms, electromyography and other analog hardware can be connected. For comprehensive research, special systems of 8, 10, 100 Hz and even higher frequency cameras are used; nevertheless elementary research can be conducted using 2-4 semi-professional digital cameras. The motion of up to several tens of labels (from 3 to 45 mm in diameter) is recorded. By selecting labels of the necessary size and distance of the measurement both relatively high motion amplitudes (such as in jumping) and relatively low motion amplitudes (such as of finger motion, jawbone motion and similar) can be measured. For jump analysis the motion trajectories of control points, the duration of phases of the jump cycle and take-off force can be directly measured [2-5]. The constructed 3D model of the lower part of the human body for the simulation of high jump enables not only investigation of parameters of the jump but analysis of the forces acting in muscles and joints as well. The model enables determination of the most important muscles for jump performance and consequently allows us to make recommendations for sportsmen seeking to improve jump height. The developed methodology can also be applied for the analysis of other types of movements in sports. We determined that the current, most frequently applied methodology of jump height determination from darting time is not suitable and should not be used for research into jump height. The calculations and research we performed allows an explanation of the stochastic character of the ground reaction force curve which is frequently recorded when performing high jumps.
A decrease in the vertical height of a jump indicates a decrease in muscle contraction capacity since jump height also depends on the magnitude (intensity of muscle capacity) of power achieved. Maximum muscle capacity is indicative of the maximum rate of ATP hydrolysis. Muscle ability to develop force depends on gender, muscle mass, the type of muscle fibers and muscle contraction type (isometric, concentric or eccentric) [6-9]. It is believed that men prevail in force over women because of their greater muscle mass and muscle capacity, as well as due to the fast muscle fibers of the II B type prevailing in male muscles, and that might be one of the reasons why men possess greater muscle force and muscle capacity. The muscle force of women during physical loading, however is less subject to fatigue than that of men [6, 7], due to their greater number of muscle II B fibers (I – 13%, II A – 34 % and II B – 53 % of muscle fibers accordingly) in men’s m. quadriceps femoris. A biopsy study of muscle fibers [7] established the following distribution of muscle fibers according to the three types in the m. quadriceps femoris of men, namely, type I – 14 %, II A – 30 % and II B – 56 %.
2. The factors influencing human jumping and their parameters
Structural basis of a human like of other vertebral living beings is his support locomotion system which consists of bones connected by mobile or immobile joints and soft tissues. This system is somewhat analogous to the supporting structures of common mechanical systems with the main difference that it changes in time. When a human organism grows, matures, and ages both geometrical dimensions and properties of structural components of the mentioned system change – mechanical properties and dimensions of bones and soft tissues; mobility and elasticity of the joints, etc. Body constitution of a certain human, his physical abilities depend greatly on the parameters of support locomotion system. As the main parameters characterizing the mentioned system can be easily measured, such measurements are named as anthropometric measurements the accumulation and systemizing the data of which was started in middle ages. Usually the main attention is paid to the measurements of general anthropometric parameters of the whole organism and for the determination of geometrical parameters of separate parts of the body (most frequently – skull, limb bones and other). Anthropometric data of a human are obtained using standardized methodologies of measurements, mainly limiting by height, weight measurements and calculation of the body mass index.
Recently for the analysis of human movements mainly a complex of means is used – very often together with computerized 3D view analysis systems by which the trajectories, velocities and accelerations of control points on the human body are determined by recording the human motion, i.e. by optical methods which compete more and more significantly with mechanical and electrical methods as they allow analyzing movements without direct contact with the body. Special labels perfectly reflecting the beams of infrared and visible spectrum are attached to characteristic points on the body and the human in motion is recorded (Fig. 1(a)). Information on the motion of characteristic points is synchronically sent from all recording cameras to computer.
Fig. 1Motion analysis by 3D view analysis system: a) recording of human motion by special facilities, b) computer model, c) example of motion analysis
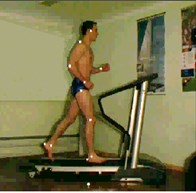
a)
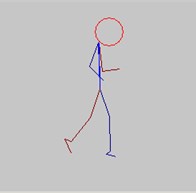
b)
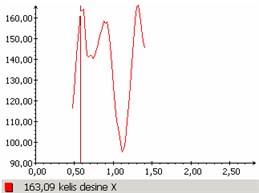
c)
Such methods are used for human gait analysis and the analysis of movement characteristics in different branches of sports. Human movements can be recorded and analyzed even by a single camera simply recording the analyzed subject and later reconstructing the recorded view in a slowed-down manner. However, more usually 3D motion analysis systems (such as Vicon, Qualisys, Simi, and Ariel among others) consist of 1-8 stationary and mobile high velocity digital cameras, a device for information transfer from cameras to computer and special software (such as Visual 3D or Simi) for the acquisition of measurement information of the motion parameters. For a more comprehensive analysis than the systems described, synchronously operating force platforms, electromyography and other analog hardware can be connected. For comprehensive research, special systems of 8, 10, 100 Hz and even higher frequency cameras are used, nevertheless elementary research can be conducted using 2-4 semi-professional digital cameras. The motion of up to several tens of labels (from 3 to 45 mm in diameter) is recorded. By selecting labels of the necessary size and distance of the measurement both relatively high motion amplitudes (such as during jumping) and relatively low motion amplitudes (such as finger motion or jawbone motion) can be measured. For jump analysis the motion trajectories of control points, the duration of phases of the jump cycle and the take-off force can be directly measured.
With the application of special software which enables automatic or semi-manual construction of a simplified model of a moving object (Fig. 1(b)) on the basis of measurement results, a more complicated analysis can be performed – the laws of joint folding angle variation, motion laws of the body segments, the laws of force change in the joints and similar parameters can all be determined (Fig. 1(c)).
3. The main stages of movement analysis methodology
For the analysis of human high jump (movements) combining the data of calculations and experimental research the system of 3D movement analysis Qualisys ProReflex which consists of four 400 Hz cameras and force measurement platform, software Qualisys Track Manager 3D (QUALISYS, Sweden) was used (initial data for human jump modeling was obtained by these means).
Special labels were attached to characteristic points of a human for measurements. They should be attached exactly to those points which correspond the control points of motion modeling software. The trajectories of control points obtained during recording later are used at computer modeling.
Experimental facilities QUALISYS for the analysis and measurements of movements equipped with four high speed digital cameras arranged in a certain order around the object under investigation are used to accumulate data on movements of a person performing high jump by recording the jump process (the change of coordinates of control points in 3D reference system) (Fig. 2). Information from the cameras is digitized by special computer board and processed by special software of movement analysis. Movement in 3D space is automatically recognized (Fig. 3) and followed forming a moving model of a human on computer screen.
Fig. 2Experimental facilities for the analysis and measurements of movements
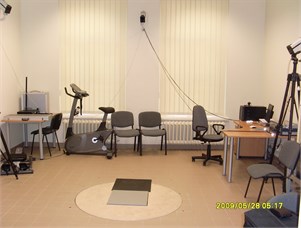
Fig. 3virtual space in which measurement of the movements is performed
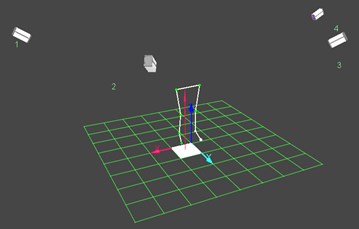
The subject under investigation had to perform jumps of two types: 1) maximum jump from an initial standing position, with the subject trying to achieve the highest possible jump, 2) maximum jump from an initial standing position, with the subject trying to perform the jump as fast as possible. These tests are commonly used to evaluate a sportsman’s jump force and the physical abilities related to it (dynamic force, spring, coordination).
Each subject had to perform a ten-minute warm-up and then perform jumps of maximal height and speed from an initial position with 90 degree bended knees, torso in vertical position and hands on hips. Each performed 5 jumps, with a 30-second interval between jumps. Jumps which failed to meet these performance criteria were not evaluated.
Table 1The techniques of vertical jumps and its description
Description | Jumping techniques | |||||
Control jumps | The jump is performed with a squat up to a right angle (some 90°) through the knee joints; hands on the waist; the angle is controlled visually | ![]() Initial position | ![]() Squat during push-off | ![]() Flying phase | ![]() Amortization phase | ![]() Final position |
Eccentric concentric jumps | The drop jump is performed from a platform 40 cm high with a squat up to a angle 90°or 135° through the knee joints with immediate maximal rebound; hands on the waist; the angle is controlled visually | ![]() Initial position | ![]() Stepping off the platform | ![]() Amortize phase | ![]() Flying phase | ![]() Final position |
The human movement analysis was accomplished in two stages: in the first stage the experimental research was performed and the side model condition obtained; in the next stage a computer-based human model was created and calculations were performed. The main stages of this methodology were as follows:
1) By recording the high jump of a human subject, using the system for measurement and analysis of 3D movements, the trajectories of control points, the laws of joint angle changes, the laws of the movements of body segments, as well as velocities and accelerations can all be determined and are then stored in the database.
2) Applying the biomechanical analysis software according to the anthropometric data of a human subject (height, weight, body proportions) under analysis, a computer biomechanical model which corresponds to the support locomotion apparatus including absolutely rigid bones, and joint elements of certain stiffness and damping as well as movement-limiting elements is constructed with muscles actuating the model.
3) On the basis of biomechanical model solving the problem of inverse dynamics (body movement benchmark trajectory or joint angle variations are indicated) the muscles activity regularity enabling experimentally measured model movement is determined.
4) Evaluation of height of vertical jump. The subjects were acquainted with the technique of performing the jump and every subject could try the technique of jumping. The contact platform (60×60 cm) with electronically fitted time – measuring device was used. Jump height was indicated by duration of flight phase [10].
The techniques of performing vertical jumps and its description are presented in Table 1.
4. Results
Support reaction force (Fig. 4), were registered during high jump.
When subjects performed high jumps and fast jumps, curves of two types were registered:
1) Those in which one peak exists in the force phase before the subject loses contact with the platform (Fig. 5 fast jump);
2) Those in which two peaks exist in the force phase before the subject loses contact with the platform (Fig. 5 high jump).
From the coordinates of control points obtained, the folding angles of hip, knee and ankle can be calculated (Fig. 6).
Fig. 4x, y, z components of support reaction force
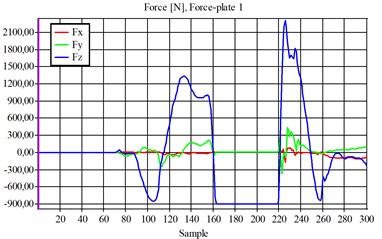
Fig. 5Force – time dependency during jump
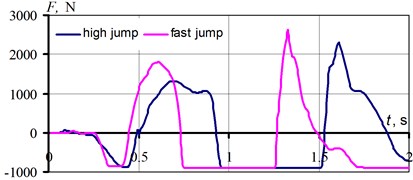
Fig. 6Folding angles of the main joints of the leg during jump
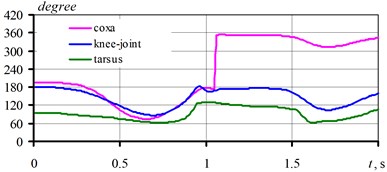
a) High jump
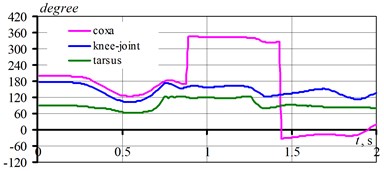
b) Fast jump
Applying biomechanical analysis software according to the subject’s anthropometric data (Table 2) allows creation of a computer-based biomechanical model coincident with the subject’s lower body supporting and movement apparatus – bones, joints, and muscles which are used for movement completion (Fig. 7). For jump analysis such a model encompasses: pelvic bone, femur bones, shinbones, splint-bones and foot bones, connective hip, knee and ankle muscles and main lower limb muscles: muscles that move the thigh, muscles that move the leg, and muscles that move the foot and toes.
Fig. 7Lower body part computer – based biomechanical model for jump analysis (bones, joints and muscles)
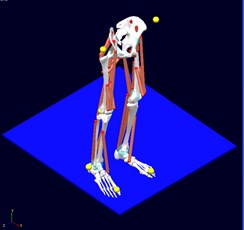
Table 2LifeMOD in terms of computer
Parameters | Remarks |
$------------UNITS | Measurements |
[UNITS] | |
LENGTH ='millimeter' | Height, mm |
FORCE ='newton' | Force, N |
ANGLE ='degrees' | Angles, degrees |
MASS ='kg' | Mass, kg |
TIME ='second' | Time, s |
$------------ANTHROPOMETRIC_DATA | Anthropometric data |
[ANTHROPOMETRIC_DATA] | |
SUBJECT_NAME = 'gal3a' | Subject name (code) |
GENDER = 1.0 | Gender (1 – male, 2 – female) |
TOTAL_BODY_HEIGHT = 1900 | Height, mm |
TOTAL_BODY_MASS = 90.00 | Mass, kg |
AGE = 276 | Age, months |
HANDS = 1 | |
NOHAT = 1 | |
$------------MARKER_SET | Marking |
[MARKER_SET] | |
TYPE = 'Davis' | |
T4= 'OFF' | OFF – inactive markers |
RSHO= 'OFF' | ON – active markers |
RASIS= 'ON' | |
RFEMW= 'OFF' | |
RFEMC= 'ON' | |
RLATM= 'ON' | |
LASIS= 'ON' | |
LFEMW= 'OFF' | |
LFEMC= 'ON' | |
LHEEL= 'OFF' | |
L2MET= 'ON' | |
SHFT= 'OFF' | |
$------------MOTION_DATA | Movement data |
[MOTION_DATA] |
Those segments which are not used in modeling the jump are deactivated, the degree of joint flexibility, which does not greatly influence the motion, is fixed (for example the knee joint can be bent only in the sagittal plane), the remaining components are modeled as passive elements, given certain values of angular rigidity and suppression (Table 3).
To solve the problem of dynamics concerning motion of a model corresponding to a human, the trajectories of benchmarks in the model were indicated and the performance laws of the above-mentioned muscles were derived in order to ensure they were determined by motion measurements.
Fig. 8 shows LifeMOD dynamic analysis and MC reaction power during high and fast jumps.
Fig. 9 and Fig. 10 depict variation of the main leg muscle forces during test jumps (left and right leg).
Fig. 9Hip Iliacus muscle: a) variation during the jump, b) left leg, c) right leg; 1 high jump, 2 fast jump
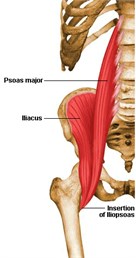
a)
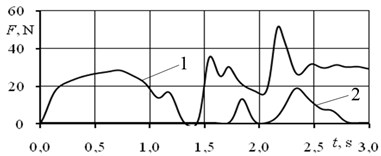
b)
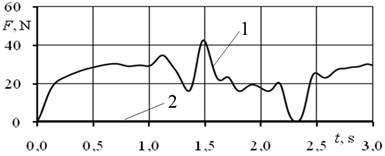
c)
Fig. 10Sacral gluteus maximus: a) variation during the jump, b) left leg, c) right leg; 1 high jump, 2 fast jump
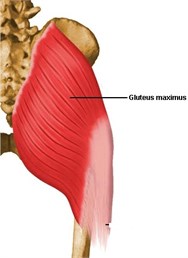
a)
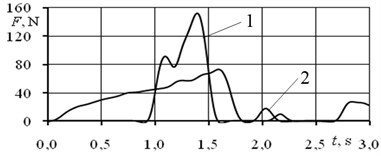
b)
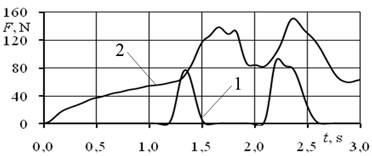
c)
Table 3Activated joint parameters
Joint | Axes* | Angular rigidity N∙mm/degrees | Angular suppression, N∙mm∙s/degrees | Freedom interval, degrees | Angular rigidity on the verge of movement freedom N∙mm/degrees |
Hip joint | 100 | 1000 | 50-120 | 106 | |
Hip joint | 100 | 1000 | 30-30 | 106 | |
Hip joint | 100 | 1000 | 60-60 | 106 | |
Knee joint | 100 | 1000 | 160-10 | 106 | |
Knee joint | Fixed freedom degree | ||||
Knee joint | Fixed freedom degree | ||||
Ankle joint | 100 | 1000 | 50-120 | 106 | |
Ankle joint | Fixed freedom degree | ||||
Ankle joint | Fixed freedom degree |
Fig. 8Dynamics analysis: a) LifeMOD (yellow movement forming markers), b) MC reaction power; 1 high jump, 2 fast jump
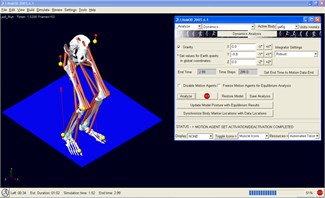
a)
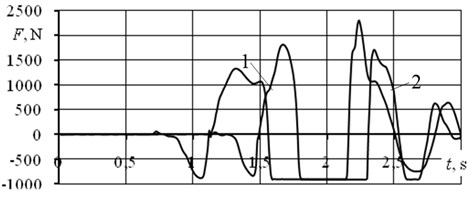
b)
5. Conclusions
From the regularities of the high jump we determined that when performing a high jump the preparation time is significantly greater than that for a fast jump. In the case of a high jump, the stochastic character of force dynamics is evident at the take-off phase. The trajectories of leg joint movements have an essential influence on force unevenness. We determined that the activity of the hip muscle and the central double shin muscle is up to 50 % higher during fast jumps and the activity of the side double shin muscle is up to 40 % higher during high jumps. Applying our 3D mathematical model of the human support-locomotion system we determined the influence of various muscles on jump height. We found that the forces developed by the muscles of each leg during jumping differ by up to 10 %. From computer analysis of jumps of different types, different muscle activities were determined during high jumps and fast jumps.
References
-
Aragon L. F. Evaluation of four vertical jump tests: methodology, reliability, validity, and accuracy. Measurement in Physical Education and Exercise Science, Vol. 4, Issue 4, 2000, p. 215-228.
-
Blanksby B., Nicholson L., Elliott B. Biomechanical analysis of the grab, track and handle swimming starts: an intervention study. Sport Biomechanics, Vol. 1, Issue 1, 2001, p. 11-24.
-
Derrick T. R., Caldwell G. E., Hamill J. Modeling the stiffness characteristics of the stiffness characteristics the human body while running with various stride lengths. Journal of Applied Biomechanics, Vol. 16, 2000, p. 36-51.
-
Haguenauer M., Legreneur P., Monteil K. M. Vertical jumping reorganization with aging: a kinematic comparison between young and elderly men. Journal of Applied Biomechanics, Vol. 21, 2005, p. 236-246.
-
McLean S. P., Holthe M. J., Vint P. F., Beckett K. D., Hinrichs R. N. Addition of an approach to swimming relay start. Journal of Applied Biomechanics, Vol. 16, 2000, p. 342-355.
-
Henriksson-Larsen K. Distribution, number and size of different types of fibers in whole cross-sections of female m. tibialis anterior: An enzyme histochemical study. Acta Physiologica Scandinavica, Vol. 123, 1985, p. 229-235.
-
Simoneau J. A., Bouchard C. Human variation in skeletal muscle fiber-type proportion and enzyme activities. American Journal of Physiology, Vol. 257, 1989, p. 567-572.
-
Kanehisa H., Okuyama H., Ikegawa S., Fukunaga T. Sex difference in force generation capacity during repeated maximal knee extensions. European Journal of Applied Physiology, Vol. 73, Issue 6, 1996, p. 557-562.
-
Pincivero D. M, Coelho A. J, Erikson W. H. Perceived exertion during isometric quadriceps contraction: A comparison between men and women. Journal of Sports Medicine and Physiology Fitness, Vol. 40, Issue 4, 2000, p. 319-326.
-
Bosco C., Luhtanen P., Komi P. V. A simple method for measurement of mechanical power in jumping. European Journal of Applied Physiology, Vol. 50, Issue 2, 1983, p. 273-282.